Dima_online_handouts.ppt
Rational crystal design: supramolecular chemistry and
molecular recognition in molecular solids
In particular the formation of cocrystals
How does
a molecule become
a material?
Supramolecular chemistry
studies the way in which the molecules join, interact and assemble to form larger assemblies. which eventually become objects in our everyday life
studies and creates objects composed of ca. 1-1,000 molecules
Crystal engineering
deals with systematic construction of crystal structures, using molecules as
building blocks. ‘bricks'
Impact on drug delivery and tablets
Why are the tablets
Why big litigation
Lipitor: Annual sales >$13 million per annum
Why are the numbers so large?
What do we know/understand about the
process of crystallization?
Main point, therefore, is: understanding molecular
Solids are (in our case) built up of molecules.
Molecular properties are largely determined by functional groups, e.g.:
Solid state properties will depend, however, on the nature of the solid that is formed.
the molecular
the structure of
solid-state properties
structure
molecular assembly
of a material
What do we mean by crystal form?
There are two general uses of the words "crystal form". One looks at the
external shape of the crystal while the other relates to the
internal arrangement of the molecule in the solid.
A molecular
view
differences in the internal architecture of the crystal:
paracetamol form I
paracetamol form II
A macroscopic view
differences in crystal habit or shape:
Level 1: Is there order within the crystal?
The first step in understanding molecular solids is in elucidating the organisation of the molecular assembly.
The solid state is
crystalline - 3-dimensional
- "random"
interconversion is possible
between structures
Level 2: Variations of the order
For a particular building block (molecule) there are many potential ways of solid-state ordering (polymorphs):
crystalline form
polymorph
1
polymorph
2
polymorph
3
polytypes
a
herringbone structure
Typically, only one polymorph is stable. The rest are metastable and may convert to the stable one.
Level 3: More than one type of species in the crystal
New crystalline forms are also achieved through formation of multicomponent
systems e.g. salts, cocrystals, hydrates, solvates, solid solutions, mixed crystals
cocrystal
Multicomponent crystals
= building block molecule
= salt former molecule
= cocrystal former molecule
= building block analogue
solid solution
Supramolecular chemistry
Supramolecular chemistry is the chemistry of the intermolecular bond based on the underlying theme of mutual recognition.
1987 Nobel prize for Supramolecular Chemistry:
Jean-Marie Lehn, Donald J. Cram and Charles J. Pedersen
J. M. Lehn
Angew. Chem. Int. Ed. Engl. 1988,
27, 89-112.
What are intermolecular bonds in a crystal?
Intermolecular bonds
Typical strength ≈ 10-15 kcal mol-1
A certain degree of directionality is required.
Hydrogen bonds (H-bonds)
Halogen bonds (X-bonds)
Molecular assembly via intermolecular bonds
Molecules within the crystal join through these non-covalent interactions to form molecular assemblies: supermolecules
Lehn: "Supermolecules are to molecules and the intermolecular bond as molecules are to atoms and the covalent bond."
polystyrene: a covalent
benzamide: crystallises from water as
hydrogen-bonded non-covalent polymeric
The entire benzamide crystal is in a sense a giant supermolecule!
A crystal as a product of supramolecular synthesis
During crystallisation of an organic solid, patterns of intermolecular interactions are established. A type of synthesis strategy. Indeed we will use the description synthons.
Analysis of crystal structures reveals that some patterns are frequently encountered:
These robust patterns of interactions are supramolecular synthons
So we can say: supramolecular synthesis through
Supramolecular synthons can be utilised to design a supramolecular synthesis, like traditional synthons of organic chemistry
The product of such a supramolecular synthesis is a two- (or more) component crystal: a cocrystal
The place of supramolecular chemistry
Understanding supramolecular chemistry is central in controlling
crystallisation and the discovery of
new solid forms
Both areas are important in the practical application of organic solids as functional materials
Important applications are in polymorphism and constructing of cocrystals with technologically important properties such as:
photo- (or thermo-) switching behaviour
reactivity
conductivity
Of particular importance are
pharmaceutical solids: the ability to
control polymorphism and improve solid forms of an active
pharmaceutical ingredient (API) would considerably shorten the
manufacture time and reduce costs!
Polymorphism of organic solids
Polymorphs can have very
different properties:
Physico-chemical: melting point, stability/reactivity, solubility, density, vapour
pressure
Mechanical: hardness, particle flow, tabletting, compactability
Spectroscopic: UV/visible (colour), IR and Raman, solid-state NMR
Pharmaceutically related: different bioavailability, different formulation
properties (e.g. flow, compaction), interactions with excipients in a tablet
Identifying and controlling polymorphic form is a major part of getting drug approval by drug licensing authorities (e.g. FDA in the US). And part of many steps in the development process.
Solid Form Selection – flow diagram of the stages in
developmentof a new drug for the marketplace
Process Development
Preformulation Formulation
Discovery of molecule
Rational selection of solid form of drug for development
Polymorphism of organic molecular solids
Polymorphs of organic solids are obtained by rearranging much weaker non-covalent forces: van der Waals interactions or hydrogen bonds
Molecular crystals are expected to exist in a larger number of polymorphs that are more readily interconvertable
Conformational polymorphism: polymorphism accompanied by changes in
molecular conformation
Example of ROY =
Red Orange and
Yellow polymorphs found initially
Conformational polymorphs have different levels of coplanarity between benzene and thiophene rings
This results in colour differences, due to different extents of delocalisation
Polymorphism of molecular solids: ROY
Now known polymorphs:
Red prisms (
R)
Yellow prisms (
Y)
Orange Plates (
OP)
Orange Needles (
ON)
Yellow Needles (
YN)
Orange-Red Plates (
ORP)
7th highly unstable form
The energy differences between polymorphs are very small, they can nucleate from
the same solution:
concomitant polymorphs
If the crystallisation and isolation are rapid enough,
concomitant polymorphs can
be separated
Hilden, JL et al Crystal Growth and Design (2003) XF#224
Formation of ROY polymorphs
Yellow prisms (
Y) is the most stable form of
ROY
At high supersaturations, nuclei of other forms in addition to
Y can form
Typically, they would dissolve, but high supersaturations enable their growth along with the stable form
Growth of metastable polymorphs: possible mechanism
Nuclei of less stable polymorphs, that would normally dissolve, can keep growing in high supersaturation regimes
High supersaturation
Polymorphism control
Instead of inducing conditions for the nucleation of new polymorphs, polymer materials can be used as "artificial (insoluble) nuclei" to seed the crystallisation reaction
This "
seeding" strategy allows the control of polymorphism and:
1)
avoids unwanted polymorphs and 2) allows the formation
of new polymorphs
Discovery of new polymorphs
Polymer heteroseeding has led to the discovery of two new polymorphs of
sulfamethoxazole, a pharmaceutical compound
Polymorphism in this case results in differences in the hydrogen-bonded framework, rather than conformation.
Polymorphism control by
pseudoseeding (heteromolecular seeding)
Crystals of compounds with molecular shape resembling the target molecule provide potential "blueprints" for new polymorphs.
Braga, D.
et al.
Topics in Current Chemistry,
1998,
198, 264.
"Jumping crystals" and mechanoluminescence
Oxitropium bromide:
Thermally-induced change in
crystal structure results in violent
jumps (up to several centimeters)
Two polymorphs of a boron-based
cyclic compound have different UV-fluorescence properties and can be
transformed by scratching and gentle warming
Compaction and making tablets
Example of paracetamol: 4-(acetylamino)phenol
Two established polymorphs:
1 and
2
Hydrogen-bonding patterns are very similar in both
easily obtained from
will form tablets,
solution but does not
but not easy to obtain
and easily form tablets
Compaction and making tablets
Form
1 contains corrugated layers, that are not easily compressed as flat
layers of form
2.
Unfortunately, form
2 is not stable and commercial
product is form
1 with lots of binding material
Patenting – not the molecule but the crystal!
Ranitidine hydrochloride
A very effective antacid
Drugs of this type are typically used to treat both duodenal and gastric ulcers
At one time Zantac was earning $2 billion per annum.
GSK (UK) vs.
Novopharm (Canada)
Major legal dispute over patents: not because of pharmacological reasons or
the identity of the molecule – but because of the structure of the crystals
polymorphic form!
Polymorphism in Molecular Crystals, J. Bernstein, Oxford Science Publications
Patenting – not the molecule but the crystal!
Conformational polymorphism
The two forms of Ranitidine HCl are
conformational polymorphs
conformational difference + disorder
Polymorphism is
helped by the flexibility of the molecule - an illustration
of the problem that affects most modern high molecular weight drugs!
Balance between intra and intermolecular forces.
Bioavailability problem – new polymorph suddenly appeared
Norvir (Ritonavir) HIV protease inhibitor from Abbott Laboratories
Initial sales of the drug were based on the properties of "Polymorph I"
In 1998: "We have encountered an undesired formation of a crystalline structure "Polymorph II" that effects how the capsule form dissolves"
Sales ceased while new formulation developed and FDA approval obtained.
In pharmaceutical industry, it is becoming crucial to understand how
molecules interact with (recognise) each other within the crystal
Most common methods of solid-state characterization
Diffraction methods: X-ray single crystal X-ray diffraction
X-ray powder X-ray diffraction (PXRD)
Spectroscopic methods:
FT IR, Raman spectroscopy, Terahertz spectroscopy
NMR spectroscopy
Thermal methods:
Thermogravimetric analysis (TGA)
Differential scanning calorimetry (DSC)
Differential thermal analysis (DTA)
Typically, diffraction and spectroscopic methods are non-destructive, whereas thermal analysis often leads to the decomposition of the sample
X-ray diffraction on a single crystal
The scattering of X-rays on a crystal can be mathematically described as the reflection of X-ray off different crystallographic planes
The diffracted beams must be in constructive interference, hence X-ray reflections are observed only for some values of θ:
2
d sin
θ =
nλ (Bragg's law)
d = spacing between a set of crystallographic planes
θ = difraction angle
λ = X-ray wavelength
n =integer
Intensity of diffracted radiation
Since there are many planes intersecting the unitcell, X-ray diffraction produces numerous reflections that are distributed in space around the crystal
Each reflection is defined by a characteristic set of Miller indices (
hkl) of the reflecting plane
Measuring the positions of reflections allows determining the symmetry and shape of the unit cell
Structure solution using powder X-ray diffraction
Powder X-ray diffraction (PXRD) relies on the same principle as single crystal diffraction, but the sample is a collection of randomly oriented crystallites
X-ray diffraction results in rings of diffraction around the sample
The X-ray detector moves through each ring, providing a diffractogram
Structure solution using X-ray powder diffraction
The two-dimensional information obtainable using single crystal X-ray diffraction is condensed into one dimension using PXRD
Significant overlap of peaks makes it difficult to extract structural information – there are two approaches:
1) Extracting individual intensities, followed by structure
solution and refinement
2) Building a model structure and fitting the experimental and
calculated diffraction patterns
Indexing of the PXRD pattern
The first step in structure determination from PXRD data is always indexing of the powder pattern: each reflection is assigned a Miller index and the unitcell is defined:
PXRD pattern of a BCC material
This can readily be accomplished for cubic structures:
sin2
θ =
λ2(
h2 +
k2 +
l2) / 4
a2
The plot of sin2
θ against (
h2 +
k2 +
l2) gives information on
a
once all the peaks have been indexed, they can treated as individual reflections, similar to single crystal reflections, proceeding to structure solution
Building a model structure
Most structures are too complicated to allow easy indexing of the powder pattern or subsequent extraction of individual intensities
The alternative approach is to generate random unitcells, taking the most common values of
a,
b,
c (between 4 and 35 Å) and
α,
β, γ (between 90 and 120 o)
The crystal system and the internal symmetry of the crystal (space group) can also be explored, trying the most common ones first:
Generating possible structures
After a suitable unit cell has been recognized, it is then filled with molecules: thousands of trial structures are thus generated:
Refining the structure
Each generated structure is validated by comparing its calculated PXRD pattern with the experimental one
When a suitable structure has been found, it is then fitted to the experimental diffraction pattern using Rietveld refinement
Identification of solid phases using powder X-ray
diffraction
The lack of order in an amorphous phase makes it "invisible" to this method
Note the use of the simulated i.e.
theoretically expected pattern
Terahertz (THz) radiation
It is non-ionising, sensitive to water content and in particular it measures intermolecular vibrations.
So unlike IR and Raman it will be very sensitive to crystal packing effects rather than intramolecular vibrations
1010 1012 1014 1016 1018
Lattice dynamics and THz spectroscopy
(courtesy of Graeme Day, Univ. Cambridge)
Carbamazepine,
form I:
Carbamazepine,
form III:
G.M. Day
et al, J. Phys. Chem. B, 110, p.
447-456 (2006)
Carbamazepine Form III
a) 37 cm-‐1
Experiment vs Simula7on
b) 50 cm-‐1
c) c) 78 cm-‐1
wavenumber / cm-‐1
G.M. Day
et al, J. Phys. Chem. B, 110, p.447-456 (2006)
Crystal engineering
We have seen examples of the possibility designing crystal structures to control
solid state property.
This corresponds to the design of materials at the molecular level, if you wish
nanotechnology
An older term is crystal engineering:
"
Understanding of intermolecular interactions in the context of crystal packing and in the utilisation of such understanding in the design of new solid with desired physical and chemical properties."
Some strategies in crystal engineering
Five approaches are:
1)
polymorph control - it is difficult to achieve and polymorphs have a bad
habit of rolling down the energy landscape to form a thermodynamically stable
one!
2)
salt formation - mostly a trial-and-error technique, heavily used in
pharmaceutical industry, as it efficiently increases
aqueous solubility of compounds
3)
formation of solid solutions - forcing a molecule to adopt a lattice site in the
crystal of a different compound.
4)
use of steering groups - specific groups are introduced onto the parent
molecule, so as to steer it into a preferred solid-state structure. Examples:
hydrogen bonding, Cl.Cl and π.π interactions
5)
formation of cocrystals - most recent and closest to the ultimate goal of crystal
engineering, utilises
supramolecular synthons to rationally construct
supramolecular structures
What is a co-crystal (cocrystal)?
One definition: A multicomponent crystalline solid, composed of more than one type of neutral molecule. Comparison with organic salts.
Co-crystals have a
stoichiometric composition - there is specific recognition
between molecules that constitute a co-crystal: some identifiable interactions.
Bird and fish are
identical shape,
in shape: only in a
they fill space in
1:1 ratio do they
any ratio (alloy).
fill space fully
Designing cocrystals
As for single component crystals at the simplest level, co-crystals can be obtained by packing molecules to fill space most efficiently
According to Kitaigorodskii, molecules will always pack so as to minimise empty space
"Nature abhors a vacuum"
For "badly-shaped" molecules, this can be achieved by adding extra building blocks: forming co-crystals based on shape-fitting
Supramolecular synthons in cocrystal design
Specific interactions between molecules can be achieved by using supramolecular synthons: robust motifs of intermolecular forces, established by database mining
Most popular synthons involve O-H.O, O-H.N, N-H.N and N-H.O hydrogen bonds
Directionality of hydrogen bonds
Directionality of supramolecular synthons
Overview of the
CSD indicates
preference of O-H.O bonds for
linearity - especially after the "cone
correction"
Robust supramolecular synthons
Supramolecular synthons enable cocrystal synthesis by design because they are robust to changes in molecular size or shape - similar to synthons of organic synthesis:
Generation of finite (or discrete) assemblies
Generation of infinite assemblies
We can also design infinite chains, sheets and cages using the acid dimer synthon:
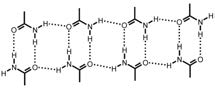
Amide-amide synthon
Formation of amide chains: a persistent motif in amide crystals. Occurs in both polymorphs of benzamide:
Describing the geometry of supramolecular synthons
Supramolecular synthons are usually described using a "graph-set" notation. Identify the h-bond arrangement, then determine:
d = the number of hydrogen bond donors
a = the number of hydrogen bond acceptors
n = the number of atoms in the motif
R22 (8) R is for "Ring"
Other motifs in graph set analysis
Comparison of homo- and heterosynthons
The examples of carboxylic acid dimers and amide chains are examples of homosynthons: they correspond to self-assembly of identical functionalities
For the design of cocrystals much more interesting are heterosynthons: recognition motifs involving different functional groups. These will encourage different molecules to crystallise together. Some of the better known are:
Adenine-thymine pairs
Acid-amide synthon
We can also consider synthons involving weak hydrogen
Weak hydrogen bonds can play a supporting role in a supramolecular synthon.
Such weak bonds may have more of a "steering" than bonding role. A good example is the pyridine-carboxylic acid heterosynthon
the supporting C-H.O bond forces the pyridine ring to be coplanar with the carboxylic acid moiety.
Variations on the theme
Another example of a supramolecular synthon composed of weak and strong interactions involves cocrystals of molecules like phenazine:
C-H.O bond again ensures the planarity of the molecular assembly
Metric engineering of anthracene cocrystals
Distribution of C-H donors of anthracene and O acceptors of the 3,5-dinitrobenzoic acid could support C-H.O bonding
Anthracene is missing any strong hydrogen-bonding sites - how about weak C-H donors? Geometric agreement seems likely.
The outcome of our design
The molecular recognition is indeed seen between anthracene C-H groups and oxygen atoms of 4-methyl-3,5-dinitrobenzoic acid!
The self-assembly of the acid leads to the formation of a "chicken-wire" framework into which anthracene is incorporated
This self-assembly is achieved by: 1) carboxylic acid dimer synthons
2) C-H.O bonds between nitro and methyl groups
Another view of the hydrogen bonding in these cocrystals
Anthracene is incorporated in the framework through weak C-H.O bonds.
If anthracene were a medicinal compound (drug), this could represent a storage or delivery structure from which anthracene could be readily removed!
How important is the methyl group?
The modular nature of the cocrystal allows us to use different components and
explore properties and test designs easily, without difficult synthesis!
We can explore a similar molecule:
4-chloro-3,5-dinitrobenzoic acid
Chloro and methyl groups are
similar in terms of size, around
20 Å3 each, but the -Cl groups
does not form C-H.O bridges!
We can also explore a slightly
different molecule:
3,5-dinitrobenzoic acid
The parent compound does not have the ability to form C-H.O linkages via methyl groups.
Chloro- and methyl groups provide
the same structure!
CH .O contacts can be replaced by
Cl.O contacts (Cl is polarisable) A strategy to construct isostructural solids: by duplicating a known structure
what about the case of hydrogen as the
substituent?
The space-filling effect
A cocrystal is not formed unless crystallization is done from a benzene solution!
Benzene participates in cocrystal formation, establishing C-H.O contacts to bridge the framework: a three-component cocrystal is formed
What is created is in fact a three-component cocrystal
Extend our definition to pharmaceutical cocrystals.
Definition: When at least one of the components is "therapeutically active" and the other components are "pharmaceutically acceptable" then we can refer to it as a pharmaceutical co-crystal.
Active Pharmaceutical Ingredient + Pharmaceutical co-crystal former
Pharmaceutical co-crystal
In this case both
components
are pharmaceutically
active
(1:1) co-crystal
Cocrystals are modular
Modularity (exchangeable components) allows function:
circumventing polymorphism:
Vishweshwar et al. Chem. Commun. 2005, 4601.
modifying melting points:
Friscic, Jones Faraday Discuss. 2007, 161.
increasing dissolution rates:
McNamara et al. Pharm. Res. 2006, 23, 1888.
caffeine:oxalic acid
Using co-crystals to improve drug bioavailability
Solubility: thermodynamic activity (extent of solubilisation)
Dissolution: kinetic parameter (rate of solubilisation)
For poorly soluble oral drugs, the rate tends to be the important parameter for absorption
For example: crystalline itraconazole is poorly absorbed orally and the
amorphous form is required for oral bioavailability
Sporanox® bead concept was introduced to overcome this difficulty, but presented a challenge in formulation development, and had initial problems with residual CH Cl
Can cocrystals be used as an alternative to the amorphous form?
First step: look for potential hydrogen bonding site
The triazole nitrogen atom does not interact with any neighbours in the pure solid itraconazole - suggests carboxylic acids as cocrystal formers:
Several of prepared cocrystals exhibited enhanced dissolution rates - comparable to the ones of the amorphous form
the cocrystal former (succinic acid) forms a "sandwich" of two itraconazole molecules
Result is increased bioavailability through cocrystals rather than marketing a metastable amorphous form.
Some strategies in crystal engineering
Five approaches are:
1) polymorph control - it is difficult to achieve and polymorphs have a bad
habit of rolling down the energy landscape to form a thermodynamically stable
one!
2) salt formation - mostly a trial-and-error technique, heavily used in
pharmaceutical industry, as it efficiently increases aqueous solubility of compounds
3) formation of solid solutions - forcing a molecule to adopt a lattice site in the
crystal of a different compound.
4) use of steering groups - specific groups are introduced onto the parent
molecule, so as to steer it into a preferred solid-state structure. Examples:
hydrogen bonding, Cl.Cl and π.π interactions
5) formation of cocrystals - most recent and closest to the ultimate goal of crystal
engineering, utilises supramolecular synthons to rationally construct
supramolecular structures
One of the best known solid-state photoreactions:
[2+2] photodimerization
Cinnamic acid and derivatives
Topochemical postulates: 1) Olefin groups must be parallel
2) d ≤ 4.2 Å
But even cinnamic acid exists as 3 polymorphs - lack of control!
Stereochemistry of the cinnamic acid dimer is controlled by the
arrangement of molecules in the reacting crystal:
α-cinnamic acid
head-to-tail product
β-cinnamic acid
head-to-head product
γ-cinnamic acid
Using steering groups to crystal engineer
appropriate alignment
How can we increase the likelihood of β-stacking in
Cl.Cl interactions are known to bring molecules at a
short (4 Å) separation
4-chlorocinnamic acid exists as a single polymorph, with photoactive contacts induced by Cl.Cl interactions
photodimerisation in a
β-stack produces only
the head-to-head
dimer
An alternative approach is to use π.π interactions
Attractive interactions between electron-rich and electron deficient aromatic moieties can also be utilised to align molecules for a [2+2] photodimerisation:
photodimerisation would produce only the head-to-tail dimer
Imperfection in this steering group design!
Each molecule in a stack has two potential photoreaction partners:
ideal reaction, 100% yield
reality, yield is limited to ca 70 %
Use a co-crystal approach: template-controlled synthesis
Template-controlled approach focuses on engineering a fragment of the crystal
structure, rather than engineering molecular stacks
Through the template-controlled approach photoactivity is isolated from the
molecular stack into a discrete molecular assembly
Resorcinol as a hydrogen-bonding template
The role of resorcinol as a template is enabled through the directionality of hydrogen
bonds (expected), as well as suitable conformation of resorcinol (hoped for)
cocrystal before reaction
Irradiated material after recrystallisation
Paracyclophane: a target for the supramolecular chemist
The interior of the photoactive assembly can be modified, enabling the control of solid-state reactivity independent of the size of the reacting molecules:
The exterior of the assembly (resorcinol R-group) can also be modified to fine-
tune solid-state reactivity.
Template-switching: a combinatorial strategy to avoid the imperfections of the approach
Undesirable photoactive olefin-olefin contacts in cocrystals with 5-OMe resorcinol template disappear upon switching to 4-benzylresorcinol:
Product yield increases from 60 to 100% after removing the parasitic reaction contact
A success of template-controlled synthesis approach: quantitative
paracyclophane synthesis
Through template-switching, crystal packing of photoactive assemblies is
modified by modifying the template, rather than the reactant
Further challenges for template-controlled approach
Solid-state photodimerization of conjugated olefins has never been observed in the solid state.
Expected products are unusual strained molecules composed of fused cyclobutane rings: ladderanes
Template-controlled solid-state synthesis of "molecular
Both strained frameworks are obtained in a 100% yield!
A single-crystal-to-single-crystal (SCSC) is a
homogeneous topotactic reaction:
i) The lattice of a solid product shows one or a small number of crystallographically
equivalent, definite orientations relative to the lattice of the parent crystal
ii) The reaction proceeds throughout the bulk of the reactant
Technological applications
(holographic memories; crystalline polymers)
In situ study of chemical reactions
(X-ray single crystal diffraction)
SCSC photodimerization
Note similarity to cinnamic acid
Monitoring reaction course through changes in unitcell
large structural changes - the
crystal eventually cracks
SCSC photochromism
Photochromism:
reversible, light-
induced change in the
color of the material
The transformation
must be reversible,
typically through light
or heat
Photochromism of dithienylethylenes is particularly interesting: 1) SCSC 2) Beautiful colors 3) Mechanical effect
SCSC reactions in
solid solutions
Mechanical effect resulting from small changes in molecular
geometry
Direct conversion of light into mechanical motion
[2+2] photodimerization of cinnamic acid
α-Cinnamic acid
Crystal decomposition is a result of rapid product buildup near the surface of the crystal because of strong and non-uniform absorption
Mismatch between product and reactant size and shape leads to the precipitation of product crystallites: polycrystalline or amorphous material results.
SCSC reactivity obtained by using radiation with weaker
absorption: tail-end irradiation
Tail-end absorption wavelengths penetrate uniformly in the crystal, enabling a homogeneous reaction.
hν
hν
Thus, [2+2] photodimerisation of α-cinnamic acid can be accomplished in SCSC manner
Alternatively reduce the size of the reacting crystals: SCSC
reactivity using nanocrystals
Reducing crystal size can enable SCSC reactivity
Polymerisation of 1,4-DSP usually occurs with crystal degradation (although small domains of homogeneous reactivity have been observed)
In nanometer-sized crystals the polymerisation is a SCSC process
SCSC reactivity by enclosing the reaction
By isolating the reacting molecules within a molecular host, changes in molecular shape upon reaction do not affect the overall crystal structure
Diacetylene 1,4-polymerization: reaction between suitably
oriented molecules of a dialkyne:
A different problem than cyclobutane synthesis: how do we template an array of molecules rather than just pairs!
Geometric data for many different derivatives and crystal
structures
Use of a self-assembled template
One possible approach is to recognise molecular functionalities that would assemble in the solid state to a similar periodic structure:
The end groups of the self-assembled template need to be able to interact with the end groups of the diacetylene reactant: via hydrogen or halogen bonds
A pyridine-substituted reactant
A carboxylic acid reactant
the geometry is not ideal and the compound polymerises slowly
Using halogen bonds
the product contains only iodine and carbon!
Can we design a new reaction?
Presumably, a 1,6-polymerization of a triacetylene is governed by topochemical
rules, similar to the well-established 1,4-polymerisation
Can we achieve a yet unknown reaction by aligning the molecules correctly?
A single-crystal-to-single-crystal polymerization
The 1,6-polymerisation of the triacetylene proceeds in a SCSC manner up to 70% yield to provide a highly conjugated polycarboxylic acid polymer:
How are cocrystals synthesized?
To create intimate homogeneous mixture, we might expect to dissolve in an appropriate solvent and then crystallize, filter, wash and dry.
Laborious and lots of waste solvent
The product can also be contaminated, if reactants are sensitive to solvent!
Other obstacles on the way to cocrystal synthesis
The synthesis of cocrystals is based on the synthesis of supramolecular bonds. These interactions are often susceptible to molecule-solvent interactions when constructing cocrystals from solution
binary cocrystal
ternary cocrystal
Different solubilities of the cocrystal components play a significant role in attempts to synthesise cocrystals from solution. To do so would need to determine the phase diagram.
Synthesis of cocrystals by grinding/milling
Hydrogen-bonds can be constructed through grinding
Etter, M.C. et al. J. Am. Chem. Soc. 1993, 115, 4411; Toda, F. et al. Chem. Commun. 1987, 279.
Braga, D et al. Dalton Trans. 2006, 1249
Grinding or mechanochemical synthesis provides a means to reduce the
amount of solvent used, avoid solubility and solvent competition effects
Different approaches to mechanochemical synthesis
liquid-controlled
formation of product
The presence of a "catalytic" amount of liquid can greatly enhance the scope of mechanochemical synthesis, its speed and yield!
The use of mechanochemistry for polymorph conversion
Liquid-assisted grinding can be used to achieve polymorph conversion
Grinding and solution growth provide the same product
Same cocrystal product
Solution Growth
Solid-state Grinding
2:1 Caffeine:Formic Acid Cocrystal
A new cocrystal stoichiometry is obtained by grinding
Solid-state Grinding
Solution Growth
Cocrystal Stoichiometry
Solid-state Grinding
(not via solution as yet)
Different stoichiometric ratio of
Cocrystal Stoichiometry
components in the cocrystal
Different polymorphs obtained via grinding
Solution Growth
with Grinding seeds
Solid-state Grinding
Form I (orthorhombic)
1:1 Cocrystal Polymorph
trifluoracetic acid
Solid-state Grinding
Use of seeds for
Solution Growth
solution growth after
with Grinding Seeds
Form II (monoclinic)
discovery by grinding
1:1 Cocrystal Polymorph
Cocrystals from solution
1:1 Caffeine:Glutaric Acid Cocrystals
slow evaporation
Polymorph I
Polymorph II
Concomitant polymorphs
Selective polymorph formation via liquid-assisted grinding
Caffeine:Glutaric acid 1:1 form I
(Obtained using cyclohexane)
Caffeine:Glutaric acid 1:1 form II
(Obtained using chloroform)
Cocrystallization from solution results in the formation of both polymorphs
Source: http://csacs.mcgill.ca/custom/docs/activities/classes/2012-class-friscic.pdf
management arrangements for victorian mangroves and coastal saltmarsh Management arrangements for mangroves and coastal saltmarsh are complex because of the occurrence of these vegetation types within the coastal zone, an area covered by a multiplicity of policies, statutes and by-laws and with diverse international, national, state and local influences. That complexity is further enhanced by the number of government agencies and non-government organisations that play a role in the planning and management of the coastal zone which encompasses mangrove and coastal saltmarsh environments.
Clinical Chemistry 57:4 Automation and Analytical Techniques Measurement of Hemoglobin A from Filter Papers for David A. Egier,1 Judy L. Keys,1 S. Kim Hall,1 and Matthew J. McQueen1,2,3* BACKGROUND: Stability and transport challenges make standardized protocols, and analyses were performed hemoglobin (Hb) A1c measurement from EDTA whole