Eurolab-d.de
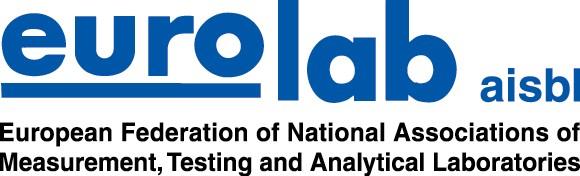
Technical Report No. 01/ 2014
Guide to NMR Method
Development and
Validation – Part I:
Identification and
Quantification
Impressum
EUROLAB Technical Report 1/2014
"Guide to NMR Method Development and Validation – Part 1: Identification and
Quantification"
May 2014
EUROLAB General Secretariat
Rue du Commerce 20-22
1000 Brussels
BELGIUM
Phone: +32 2 511 50 65
e-Mail: [email protected]
URL: www.eurolab.org
T. Schönberger, Y.B. Monakhova, D.W. Lachenmeier, T. Kuballa, Non-Profit Expert Team
(NEXT) -NMR working group Germany
NEXT-NMR-working group Germany in detail:
J. Ammon, C. Andlauer, E. Annweiler, H. Bauer-Aymanns, M. Bunzel, E. Burgmaier-Thielert, T.
Brzezina, N. Christoph, H. Dietrich, A. Dohr, O. el-Atma, S. Esslinger, S. Erich, C. Fauhl-Hassek,
M. Gary, R. Godelmann, V. Guillou, B. Gutsche, H. Hahn, M. Hahn, A. Harling, S. Hartmann, A.
Hermann, M. Hohmann, M. Ilse, H. Koch, H. Köbler, M. Kohl-Himmelseher, K. Klusch, U.
Lauber, B. Luy, M. Mahler, S. Maixner, G. Marx, M. Metschies, C. Muhle-Goll, G. Mildau, M.
Möllers, C. Neumann, M. Ohmenhäuser, C. Patz, D. Possner, I. Ruge, W. Ruge, R. Schneider, I.
Straub, C. Tschiersch, G. Vollmer, H. Wachter, S. Walch, P. Weller
Contents
1. General conditions
1.1 Acquisition parameters
1.1.1 Homogeneous magnetic field
1.1.2 Temperature stability
1.1.3 Data acquisition time
1.1.4 Pulse angle
1.1.5 Number of data points
1.1.6 Relaxation time
1.1.7 Electronic amplification
1.2.2 Phase correction
1.2.3 Baseline correction
1.3 Quantitative analysis
1.3.1 Signal integration
1.3.2 Alternative techniques
2. Identification
2.1 Single analytes
3. Quantitative NMR (qNMR)
3.1 Internal calibration
3.2 External calibration
3.3 High-precision determination of analytical standards purity
4. Parameters for validation
4.4 Limit of detection (LOD)
4.5 Limit of quantification (LOQ)
4.6 Linear range
4.7 Working range
4.9 Measurement uncertainty
Foreword
Nuclear Magnetic Resonance (NMR) spectroscopy is one of the most versatile methods of
analysis. Till the early 1970s, NMR spectroscopy was exclusively used for structure
elucidation and purity testing of synthesized compounds. Nowadays, however, the range of
successful applications covers identification and structure elucidation of organic and
biochemical molecules, precise quantitative determination of individual analytes and
multicomponent analysis as well as so-called "non-targeted screening" in combination with
different chemometric techniques [1, 2]. Since NMR is a very selective analytical tool (each
molecule has its own spectral fingerprint), not only quantification of ingredients is possible,
but also comparison, discrimination or classification of foods, beverages and other consumer
products can be achieved (e.g., authenticity evaluation, determination of origin and botanical
variety of certain products) [1]. "Non-targeted" NMR analysis allows a fast and highly
selective sample screening, from which much more information can be obtained than by using
any other analysis technique that was previously used for this purpose [1].
NMR techniques have been an integral part of scientific research for a long time and are
increasingly used in the monitoring of foods, beverages, cosmetics and pharmaceuticals. The
special criteria proposed in this report should facilitate development of any practical
application of a NMR method in these areas of applications. Furthermore, our suggestions are
in accordance with quality management (QM) system required for governmental food control
[3-5]. It should be mentioned, however, that the conditions listed in this report must always be
adapted for the specific analytical problem, the most recent QM requirement and the matrix
under investigation.
1. General conditions
For NMR analysis, the operating manuals of the respective equipment manufacturer have to
be followed and the operation must be performed by qualified personnel.
1.1 Acquisition parameters
1.1.1 Homogeneous magnetic field
To obtain meaningful NMR spectra, a homogeneous magnetic field must be achieved with
suitable shimming routines.
1.1.2 Temperature stability
A constant temperature with a maximum drift of 0.1 K has to be maintained during the
acquisition of NMR spectra.
1.1.3 Data acquisition time
The data acquisition time must be chosen so that truncation of the Free Induction Decay (FID)
1.1.4 Pulse angle
In NMR spectroscopy, pulse angles up to 90 degrees can be chosen for acquisition. The
employment of 90 degree pulse in quantitative NMR leads to the maximum signal intensity
with respect to the measurement time spent. In quantitative NMR using external calibration,
the 90 degree pulse angle is preferably used.
The determination of the pulse angle must be uniform and can be done either by using an
iterative, visual method or by automated methods [6].
1.1.5 Number of data points
In quantitative NMR (qNMR), the number of acquired data points must be large enough to
describe the shape of a signal accurately. Ideally, more than 10 data points should be above
the half of the peak height [7, 8].
1.1.6 Relaxation time
In qNMR, the longitudinal (spin-lattice) relaxation time T1 has to be considered. T1 is
different for each signal of every compound and can also vary depending on the matrix (pH,
salt content, etc.). To be on the safe side, the sum of the relaxation delay and the acquisition
time should exceed five times the highest T1 value of all evaluated signals. Theoretically, the
factor 5 will lead up to 99.0 % of the maximum signal intensity, the factor 7 will lead up to
1.1.7 Electronic amplification
The receiver gain (RG) must be set for each sample to be analyzed, so that no truncation of
the FID occurs. This is particularly important for quantitative analysis. For chemometric
methods and quantification by external calibration, it must be ensured that always the same
signal amplification is used.
1.2 Processing
1.2.1 Smoothing
The exponential multiplication is widely used as apodization function for 1D NMR spectra. In
the quantitative 1H NMR, low smoothing factors (lb, line broadening) of 0.1-0.3 Hz are
suitable. They result in an increase of the signal-to-noise (S/N) ratio without distortion of
signal intensities ratio [9].
1.2.2 Phase correction
Phase correction can be performed automatically or manually. Automatic correction may not
be sufficient for qNMR in some cases. Exact phase correction has to be ensured for accurate
1.2.3 Baseline correction
For qNMR, baseline correction is fundamental. A number of mathematical functions are
available for this purpose. It is necessary to pay attention that only the baseline itself, but not
the actual signal is corrected. In addition, partial baseline correction may be useful to
correctly evaluate signals situated near very broad signals.
1.3 Quantitative analysis
1.3.1 Signal integration
To achieve accurate integration of signals, no interference of the analyte of interest with other
signals must occur. The integral width should be chosen so that the same parts of the analyte
and reference signals are used for integration (especially with/without 13C satellites in 1H
A manual correction of the integral parameter, known as ―slope‖ and ―bias‖, should be
avoided. However, this is typically not necessary if the baseline has been accurately corrected.
1.3.2 Alternative techniques
There are other alternative methods for signal evaluation. These include, for example, the
evaluation of the signal heights [10] or signal deconvolution [11]. The necessary parameters
of these methods as well as their limitations and uncertainties are not described in this report.
However, to use these alternative methods one has to be aware of their application range and
2. Verification and identification
2.1 Single analytes
If only NMR spectroscopy is used for structure verification (e.g. comparing of sample
composition with the list of ingredients), it is usually sufficient that all the characteristic
signals are detected and assigned. The measurement of a single pulse 1D spectrum (1H or 13C
-NMR) is sufficient in most cases. For identification and verification in complex cases the
application of 2D correlation spectra (e.g. J-Resolved (J-Res), H,H-COSY, HSQC, HMBC)
might become necessary. Depending on the analytical problem it could be also helpful to
observe different nuclei (e.g. 15N, 19F, 31P).
Ideally, a spectrum of a reference material should always be recorded under the same
conditions as the analyte. In cases of spectral overlap between analyte and reference
compound, the identification can be carried out optically by stacking both spectra. Reference
spectra from databases and scientific publications can also be used for identification.
Theoretical calculations of spectra using tables or prediction programs can be used as an
auxiliary tool, but typically is not sufficient for unambiguous identification. In cases when no
reference data are available, a complementary technique should be used. Mass spectrometry is
especially suitable for this purpose [12]. The application of the complementary technique
might be unnecessary if useful a priori information about the sample is available (synthesis
2.2 In mixtures
In mixtures or in natural matrices some characteristic signals of an analyte may be overlapped
in the spectrum. Ideally, all signals of an analyte should be identified and assigned, for
example, by comparison with reference spectra.
Depending on the matrix under investigation, it may be sufficient if only single signals of a
particular compound can be clearly assigned. This is especially the case if extensive
information about matrix ingredients is available (e.g. in the case of fruit juices).
Identification of substances in mixtures can be facilitated by the application of 2D
spectroscopy (especially HSQC and J-Res) or Diffusion Ordered Spectroscopy (DOSY)
Regarding the number of required identification points no general rule exists. For example,
for sugars in fruit products one signal of an anomeric proton may be sufficient for
identification. However, for the differentiation of synthetic drugs with similar structures
almost all signals may be needed.
3. Quantitative NMR (qNMR)
Since NMR technique is a quantitative technique per se, it can be also applied for quantitative
analysis considering the requirements of DIN/ISO 17025 [5]. Many common methods in
analytical chemistry such as external quantification, internal quantification or standard
addition are also applicable for NMR [13]. A great advantage of the NMR technique is the
possibility of quantifying an analyte without reference substance by applying another
reference standard.
3.1 Internal calibration
For internal calibration a defined amount of a reference material is added to the sample
solution. The quantification is performed by comparing the integrals (or an equivalent
response) of analyte (X) and reference (Std). The analyte concentration is calculated
according to the following equation:
The numbers of protons generating the selected signals for integration
Molecular weight
The utilized reference materials must meet the following minimum requirements [9]:
• known purity
• no overlapping signals in a given matrix
• precisely weighable (non-hygroscopic / non-volatile)
• chemically inert with respect to the solvents used and the matrix analyzed
The traceability of a certified reference material has to be ensured.
3.2 External calibration
Quantification can be also carried out by external calibration. A number of different methods
are available for this purpose (e.g. coaxial tubes [14], ERETIC [15, 16], PULCON [17, 18],
Quantas [19]). In the course of method validation all features and characteristics of the
method used must be evaluated and analyzed. External calibration by a calibration curve with
different analyte concentrations can be also used [20].
Increasingly PULCON is used for quantification in chemical and biological applications [17].
This principle method can also be used for quantification of analytes in food like fruit juice,
wine or dietary supplements.
3.3 High-precision determination of analytical standards purity
The high-precision analysis (uncertainty of measurement < 0.3 %) of pure substances
demands further requirements. All parameters must be optimized in terms of precision. As the
precision is highly dependent on S/N, an extremely high S/N of more than 10,000:1 is
required for such quantification [8].
4. Parameters for validation
Parameters that have to be validated for the specific method are summarized in the following
determination of analytical standards purity (assay)
4.4 Limit of detection
4.5 Limit of quantification
4.6 Linear range
4.7 Working range
4.1 Trueness
If all important parameters are taken into account, no systematic errors should be found. For
qNMR, the proof of trueness could be performed by comparing the purities of several
certified reference materials or by the comparison with an independent method of known
4.2 Precision
Precision of the NMR method is determined by multiple measurements within a short time of
several re-weighted samples of one matrix (including sample preparation).
For the determination of laboratory precision one sample should be analyzed several times in
one laboratory by systematically changing parameters, such as operator, device or time.
If inter laboratory precision (reproducibility) is required, it can be determined by comparative
measurement of a sample in different laboratories.
4.3 Selectivity
NMR spectroscopy provides very detailed structural information and, therefore, it possesses
in principle, high specificity. However, specificity may not be achieved, if, for example,
necessary signals for identification cannot be assigned due to signal overlap.
qNMR is considered to be selective when it can be ensured that the analyzed signal does not
overlap with other components. It is sufficient to prove the specificity of the method for some
most critical examples.
If alternative methods are used for the quantitative evaluation (1.3.2), the selectivity
requirements can be met despite the signal overlap. This must be proved in each particular
4.4 Limit of detection (LOD)
Limit of detection (LOD) is an important validation parameter relevant for the identification
of an analyte. At the concentration of LOD positions and shapes of all relevant analytes'
signals in the spectrum can be visually recognized [21]. The detection limit can be determined
mathematically or visually from the spectrum. Mathematically LOD can be determined for the
relevant signal as intensity for which S/N equals 3. The visual way for LOD determination is
shown on Fig.1 on the ephedrine-HCl as an example:
Fig.1: Signals of ephedrine-HCL of 0.084 mg (bottom), 0.025 mg (middle) und 0.007 mg
(top).
It can be seen that ephedrine signals can be clearly visually identified at 0.025 mg (Fig.1).
Therefore, this amount can be regarded as the detection limit for this compound.
It should be noted that the signal intensity is dependent on the signal's shape (line width,
coupling pattern) and on the number of corresponding nuclei. Apart from that, signal intensity
is proportional to the concentration of substance (if all requirements for qNMR are fulfilled).
If common quantification routines are used (valid for any analyte in a given matrix), it is
sufficient to determine the LOD (and also limit of quantification, LOQ) for a single analyte in
a matrix. The detection limit for another analyte can be assessed from this value by
considering different signal shapes.
The limits of detection (LOD) and the limits of quantification (LOQ) can be also calculated
based on the standard deviation of the response and the slope of the calibration curve. This
procedure is also approved by the German Institute for Standardization and is regarded as a
method, from which reliable and practically meaningful values can be obtained [22]. These
calibration curves can be constructed based on the integration of specific resonances for each
compound and known analyte concentration (if matrix effect should be taken into account,
spiked solutions can be used).
Fig.2: Calibration curve for HMF in apple juice matrix near LOD/LOQ values with
95% prediction limits.
4.5 Limit of quantification (LOQ)
Limit of quantification (LOQ) is the concentration of analyte, for which the selected signal
exhibits the minimal required S/N for the given method precision [21]. The minimum
required S/N can be determined experimentally by means of multiple measurements at
different dilutions. The relative standard deviations are determined in each case. The
minimum S/N is then equal to the concentration, for which acceptable standard deviation has
been obtained. This standard deviation should be oriented on the standard measurement
uncertainty of the method (Table 1).
See also 4.4 for calibration curve method [22].
Table 1 Values of measurement uncertainty as the function of S/N as an example [23].
Relative measurement
uncertainty in %
4.6 Linear range
Due to the high dynamic range of modern NMR spectrometers the linearity is ordinarily an
uncritical parameter. The signal intensity is proportional to the number of nuclei.
The linearity of the NMR spectrometer used must be validated only once by using at least 5
different concentrations of any readily soluble substance. The chosen concentrations should
cover the working range of the method. The linearity should be evaluated by appropriate
statistical methods, such as calculation of a regression line by the method of least squares [21]
4.7 Working range
The working range of an NMR method usually starts from the LOQ (lower limit) to the
analyte solubility (upper limit) for an analyte in a given matrix. This standard procedure is
suitable for each analyte in a given matrix. For another matrix, it must be ensured that the
analyte has been completely dissolved and that no complex formation / aggregation /
degradation takes place. This can be done by visual inspection of the solution or, if insoluble
residues of other components remain in solution, through multiple measurements at different
concentrations and by comparison of actual and reference line widths of the signals of
interest. For critical analytes or in complex matrices the linearity of the working range should
be additionally checked.
4.8 Robustness
For the validation of the robustness, possible influences of different sources must be
considered and consequently examined (e.g. pH values, high salt concentrations of the sample
matrix, reactive chemicals). The robustness checks for such possible impacts on the final
4.9 Measurement uncertainty
The measurement uncertainty results from the previously mentioned performance
characteristics such as trueness and precision [24,25]. The uncertainty of measurement
predicted by the uncertainty budget must correspond to the uncertainty of multiple
determinations of concentration of analyte in sample. The method is said to be in statistical
control when this condition is accomplished
When no systematic error is detected, the measurement uncertainty is equal to the
precision. In other cases, the calculated analyte concentration should be adjusted by estimated
recovery values. For example, degradation up to 50% of spiked concentration for some
substances (acetaldehyde, acetoin, lactic acid and pyruvic acid) in apple juice matrix was
observed, leading to unacceptable recovery values. The calculated concentrations were
corrected using results from the degradation studies in water [26].
Alternatively, the measurement uncertainty can be calculated from the combination of
specific individual factors (e.g., weighing, preparation, measurement, calculations, etc.)
The uncertainty of reference material´s purity must be also taken into account when it is of the
same order of magnitude as the overall method´s uncertainty.
The confidence interval has to be reported together with the uncertainty value.
5. Literature
1. Le Gall G., Colquhoun I.J., NMR Spectroscopy in food authentication (in Food authenticity
and traceability), Woodhead Publishing, New York, 131, 2003
D.W.E.F.B.P.C.M.,
NMR-Spectroscopy for nontargeted screening and simultaneous quantification of health-
relevant compounds in foods: the example of melamine, Agric Food Chem, 57(16): 7194–
3. Irish National Accreditation Board, Guide to method validation for quantitative analysis in
chemical testing laboratories, Dublin, 2012
4. Thompson M., Ellison S., Wood R., Harmonized guidelines for single-laboratory validation
of methods of analysis (IUPAC Technical Report), Pure Appl Chem, 74, 835-855, 2002
5. International Organization for Standardization, ISO (2005) ISO/IEC 17025 General
requirements for the competence of testing and calibration laboratories, Geneva, 2005
6. Wu, P.S., Otting, G., Rapid pulse length determination in high-resolution NMR, J Magn
Reson, 176(1), 2005
7. Malz, F., Quantitative NMR-Spektroskopie als Referenzverfahren in der analytischen
Chemie, Dissertation, Berlin, 2003
8. Schoenberger, T. Determination of standard sample purity using the high-precision 1H-
NMR process, Anal Bioanal Chem, 403(1), 247-254, 2012
9. Schoenberger, T., Development and validation of a high-precision quantitative NMR
procedure for the purity determination of reference standards, Master Thesis, Idstein, 2011
10. Hays P.A., Thomson R.A., A processing method enabling the use of peak height for
accurate and precise proton NMR quantitation, Magn Reson Chem, 47(10), 819-824, 2009
11. Crockford D.J., Keun H.C., Smith L.M., Holmes E., Nicholson J.K. Curve-Fitting Method
for Direct Quantitation of Compounds in Complex Biological Mixtures Using 1H NMR:
Application in Metabonomic Toxicology Studies, Anal Chem, 77(14), 4556-4562, 2005
12. Lommen A., Schilt R.,Weseman J., Roos A.H., van Velde J.W., Nielen M.W.F.,
Application of 1D 1H NMR for fast non-targeted screening and compositional analysis of
steroid cocktails and veterinary drug formulations administered to livestock. J Pharm
Biomed Anal, 28, 87-96, 2002
13. Pauli G.F., Gödecke T., Jaki B.U., Lankin D.C., Quantitative 1H NMR. Development and
Potential of an Analytical Method: An Update. J Nat Prod 75, 834-851, 2012
14. Henderson T.J., Quantitative NMR spectroscopy using coaxial inserts containing a
reference standard: purity determinations for military nerve agents, Anal Chem, 74(1), 191-
15. Barantin, L., Le Pape, A., Aloka, S., A New Method for Absolute Quantitation of MRS
Metabolites, Magn Reson Med, 38(2), 179-182, 1997
16. Akoka, S., Barantin, L., Trierweiler, M., Concentration Measurement by Proton NMR
Using the ERETIC Method, Anal Chem, 71(13), 2554-2557, 1999
17. Wider G., Dreier L., Measuring protein concentrations by NMR spectroscopy, J Am
Chem Soc, 28(8), 2571-2576, 2006
18. Dreier L., Wider G., Concentration measurements by PULCON using X-filtered or 2D
NMR spectra, Magn Reson Chem, 44, 206-212, 2006
19. Farrant R.D., Hollerton J.C., Lynn S.M., Provera, S., Sidebottom, P.J., Upton R.J., NMR
quantification using an artificial signal, Magnet Reson Chem, 48(10), 753-762, 2010
20. Maes P., Monakhova Y.B., Kuballa T., Reusch H., Lachenmeier D.W. Qualitative and
Quantitative Control of Carbonated Cola Beverages Using 1H NMR Spectroscopy, J Agric
Food Chem, 60(11), 2778-2784, 2012
21. International Conference on Harmonization (ICH) of Technical Requirements for the
Registration of Pharmaceuticals for Human Use, Validation of Analytical Procedures: Text
and Methodology, 1996
22. Deutsches Institut für Normung, DIN 32645 Chemical analysis – Decision limit, detection
limit and determination limit under repeatability conditions – Terms, methods, evaluation,
23. Schoenberger. T, Validation of Quantitative NMR Methods, Bundeskriminalamt, 2012
24. Joint Committee for Guides in Metrology, Evaluation of measurement data — Guide to
the expression of uncertainty in measurement, 2008.
25. Magnusson, B., Näykki, T., Hovind, H., Krysell, M., Nordtest Report, Handbook for
Calculation of Measurement Uncertainty in Environmental Laboratories, Edition 3.1,
26. Monakhova Y. B., Schütz B., Schäfer H., Spraul M., Kuballa T., Hahn H., Lachenmeier
D. W. Validation studies for multicomponent quantitative NMR analysis: the example of
apple fruit juice. Accred Qual Assur, 19(1), 17-29, 2014
Source: http://eurolab-d.de/files/guide_to_nmr_method_development_and_validation_-_part_i_identification_and_quantification_may_2014.pdf
Industry: Pharmaceuticals RECOMMENDATION: HOLD Johnson & Johnson is well positioned within the global Closing Price $59.13 (6/21/10) health care market. The company uses innovative products, $66.20 (4/20/10) robust pipelines and its talented people to capture a global $54.91 (6/23/09) presence in the health care industry. Johnson & Johnson is
International Journal of Cancer A mixture of amino acids and other small molecules presentin the serum suppresses the growth of murine and humantumors in vivo ´ kos Schulcz2 and Tamas Cz€omp€oly1 1 Immunal Ltd., Cancer Research and Product Development Laboratory, H-7630 Pecs, Finn u. 1/1., Hungary2 Department of Experimental Pharmacology, National Institute of Oncology, H-1122 Budapest, Rath Gy€orgy u. 7-9., Hungary