Gregfridman.org
Plasma Chem Plasma Process (2012) 32:165–176DOI 10.1007/s11090-011-9336-x
Effect of Dielectric Barrier Discharge Treatmentof Blood Plasma to Improve Rheological Propertiesof Blood
Jin M. Jung • Yong Yang • Dong H. Lee • Greg Fridman •Alexander Fridman • Young I. Cho
Received: 19 August 2011 / Accepted: 28 November 2011 / Published online: 4 December 2011Ó Springer Science+Business Media, LLC 2011
The whole blood viscosity (WBV) is one of the major independent indicators
for the risk of cardiovascular disease, stroke, and peripheral arterial diseases. Furthermore,oxidized LDL molecules are known to cause atherosclerotic plaques in arteries, and it isone of the key components that increase WBV. The present study attempted to reduceWBV by coagulating plasma proteins and lipid molecules from blood plasma using non-thermal dielectric barrier discharge (DBD) and removing them through filtration. The DBDtreatment was found to produce coagulated particles in blood plasma. After filtration of thecoagulated particles, WBV decreased by 9.1 and 17.7% for both systolic and diastolicblood viscosities, respectively. The present results suggest that the removal of excessplasma proteins and lipid molecules might be feasible using DBD treatment.
Dielectric barrier discharge (DBD) Blood viscosity Filtration
The viscosity of a fluid represents the frictional resistance between a moving fluid andstationary wall. Blood viscosity is the inherent resistance of blood to flow and representsthe thickness and stickiness of blood. Since about 45% of blood volume is made up ofsuspended cellular particles, primarily red blood cells, the blood behaves as a non-Newtonian fluid where its viscosity varies with shear rate (i.e., the ratio of flow velocity tolumen diameter). The dynamic range of the whole blood viscosity (WBV) is relatively
J. M. Jung Y. Yang A. Fridman Y. I. Cho (&)Department of Mechanical Engineering and Mechanics, Drexel University, Philadelphia, PA, USAe-mail:
[email protected]
D. H. LeeDepartment of Mechanical Design Engineering, Engineering College, Chonbuk National University,Jeonju, Republic of Korea
G. FridmanSchool of Biomedical Engineering, Drexel University, Philadelphia, PA, USA
Plasma Chem Plasma Process (2012) 32:165–176
large, i.e., 40–450 millipoise (mP) (or 4–45 centipoise), which highlights the potentialutility of this parameter as a biomarker—to the degree that viscosity provides additionalincremental prediction of clinical outcomes and is modifiable by therapeutic modalities.
WBV has been strongly associated with cardiovascular disease stroke and
peripheral arterial disease []. Mechanical interaction between blood and bloodvessels mediated by the increase of WBV has a crucial pathogenic role in the release ofendothelium-derived mediators (NO and endothelia), thus causing subsequent vascularremodeling by activation of endothelium, initiation of inflammation, alteration of lipidmetabolism, and finally progression of atherosclerotic vascular disease –
There are a number of variables for the WBV, which include hematocrit, plasma
proteins (i.e., fibrinogen, immunoglobulin, and albumin), total cholesterol, low-density-lipoprotein (LDL) cholesterol, high-density-lipoprotein (HDL) cholesterol and triglyceride[]. In addition, the aggregation and deformability of erythrocytes critically affect theWBV , ]. Since both the plasma proteins and LDL molecules influence the aggre-gation of erythrocytes it is important to keep both levels within the respectivenormal ranges. For example, lipid-lowering statin drugs have been widely used to keep theLDL cholesterol within the normal range (i.e., 62–130 mg/dl) Statins are pow-erful cholesterol-lowering drugs in clinical practice and have a life-saving potential inproperly selected patients, particularly those with severe hyperlipidemia and atheroscle-rotic disease [, ]. Results from randomized clinical trials have demonstrated a decreasein congested heart diseases (CHD) and total mortality, reductions in myocardial infarc-tions, revascularization procedures, stroke, and peripheral vascular disease However, statins are prescribed for less than half of the patients who should receive thistherapy, unfortunately, because of fear of liver and muscle toxicity, a side effect whichremains a major impediment to the appropriate use of these drugs [].
In recent years, many new medical applications, including the sterilization of living
tissue without damage, blood coagulation, the induction of apoptosis in malignant tissues,and the modulation of cell attachment are being developed utilizing non-thermal plasmadischarges in atmospheric pressure []. It was demonstrated that selective coagulationof fibrinogen, which is one of the major viscosity determinants in blood, could be inducedby the application of dielectric barrier discharges (DBD) in air Hence it is hypoth-esized that the hemorheological properties of blood may be improved by lowering thelevels of fibrinogen and LDL molecules in blood plasma through DBD-assisted coagula-tion and the subsequent filtration of the coagulated particles from the treated blood plasma.
Hence, the objective of the present study was to investigate the feasibility of reducingwhole blood viscosity through DBD treatment of blood plasma and subsequent filtration.
Experimental Setup for DBD Treatment
The DBD system used in the present study was the identical to the one used by Kalghatgiet al. [], which was atmospheric pressure dielectric barrier discharge and is illustrated inFigs. and . The DBD system was operated with one dielectric-covered powered elec-trode and the other grounded electrode. Discharge was ignited when the powered electrodeapproached the surface of the sample to be treated at a distance of less than 3 mm, thephenomenon which depended on the waveform, duration, and polarity of the drivingvoltage []. A pulsed high voltage of 35 kV (peak to peak) with alternating polarity at
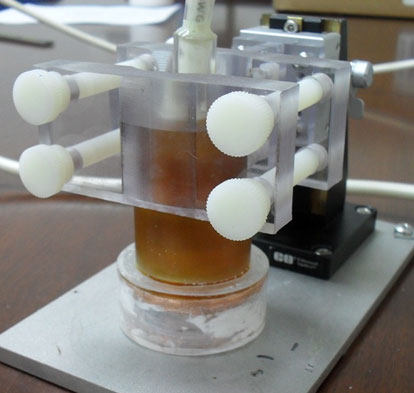
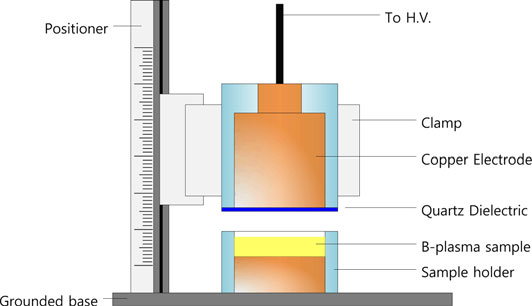
Plasma Chem Plasma Process (2012) 32:165–176
Fig. 1 Application of DBD toblood plasma sample
Fig. 2 Schematic of the experimental setup for the DBD treatment of blood plasma
1 kHz frequency was applied between the quartz insulated copper electrode and the surfaceof the sample (blood plasma) to generate DBD. The power applied to the present DBDsystem was analyzed by measuring the current passing through the discharge gap and thevoltage drop in the gap when the discharge was generated. The current and voltage signalswere acquired and recorded by a two-channel digital phosphor oscilloscope (DPS)(TDS5052B, 500 MHz bandwidth, 5 GS/s sample rate, Tektronix, Inc). The surface powerdensity which corresponded to the applied power was around 1.5 W/cm2 [,
The electrode was constructed of a 25 mm diameter copper rod enclosed in polythe-
rimide (UltemÒ). A 1 mm thick fused quartz was used to prevent an arc formation bylimiting the current in the DBD discharge. The presence of the insulating layer preventedthe buildup of high current and subsequent heating of the gas in the discharge gap so thatbiological samples could be treated without thermal damage. The gap between the bottomof the dielectric quartz glass covering the copper electrode and the surface of the blood
Plasma Chem Plasma Process (2012) 32:165–176
plasma sample was adjusted to be 2 mm using a precision vertical positioner shown inFig. To treat the sample with DBD, 4 mL of blood plasma were placed in the sampleholder (see Fig. which was composed of a 25-mm-diameter copper rod inserted intopolytherimide (UltemÒ) shell. The bottom of the copper rod inside the sample holder wasplaced on a 21 mm thick polycarbonate plate as the grounded base electrode ,
Experimental Procedure
Human whole blood of 150 mL with 1.5 mL of ethylene diaminetetraacetic acid (EDTA)as an anti-coagulant was obtained from Lampire Biological Laboratories (Pipersville, PA)in a glass bottle. Both the WBV and hematocrit for the blood sample were measured todetermine the baseline data prior to the DBD treatment. The whole blood in the 150 mL ofbottle was then transferred to several 10 mL-polyethylene tubes to prepare blood plasmafrom whole blood using a centrifuge (VanGuard V6500, Hamilton Bell Co.) with 1200 Gof relative centrifugal force (RCF) for 15 min.
The blood plasma viscosity was measured before DBD treatment for the baseline data.
Each blood plasma sample separated from whole blood was then treated with DBD for 1, 2,4, and 8 min to investigate the effect of DBD treatment on the rheological properties ofblood plasma and whole blood. Temperature change was monitored before and after theDBD treatment.
The viscosity of the blood plasma after DBD treatment was measured again with
viscometers (see below) to compare the change in the plasma viscosity before and afterDBD treatment.
In order to evaluate the change in the WBV due to the coagulated particles generated by
DBD treatment, some of the DBD-treated blood plasma samples were mixed back with theoriginal erythrocytes which had been separated in the previous step, and the WBV wasmeasured. For the last step, to investigate whether or not the WBV could be reduced by thefiltration of the coagulated particles from the DBD-treated blood plasma, some of theDBD-treated blood plasma samples was filtered using a syringe filter (25 mm, pore size0.20 lm, Nylon, Millipore) and then mixed back with the centrifuged erythrocytes, and theviscosities of the mixed whole blood samples were measured.
Here, hematocrit concentration was also measured to examine any possible plasma loss
or hemolysis during the transfer of blood, the centrifugation of blood cells, the DBDtreatment, the filtration process of DBD-treated blood plasma, and the remixing of theDBD-treated blood plasma with the centrifuged erythrocytes.
Laboratory Analyses
WBV was measured at 37°C using Hemathix viscometer (Health OnVector, Inc.) over arange of shear rates from 1 to 1,000 s-1. Detail test and viscosity calculation proceduresare given elsewhere [The viscosity of the DBD-treated blood plasma was also mea-sured using Brookfield viscometer (LV-III with CP-42, Brookfield Engineering Lab.) attwo different shear rates of 225 and 450 s-1. The temperature of the test sample atBrookfield viscometer was maintained at a constant temperature of 37°C with a constanttemperature water bath and circulator. Since some plasma volume could be lost during theDBD treatment and filtration, the WBV was normalized to a standard hematocrit of 45%using a standard hematocrit-correction method ].
Plasma Chem Plasma Process (2012) 32:165–176
Statistical Analyses
The mean and standard deviations for both whole blood and blood plasma viscosities werecalculated. WBV was reported both at the observed (native) hematocrit and normalizedhematocrit of 45% [].
The WBVs of blood samples were measured using Hemathix Analyzer at five differentshear rates of 1,000, 300, 100, 10, 1 s-1 before DBD treatment and the obtained WBVsincluding the baseline mean and standard deviation values were normalized to standardizedhematocrit of 45%. The normalized WBV of untreated blood varied from 35.38 to 301.01mP when shear rate decreased from 1,000 to 1 s-1.
When blood plasma was treated with DBD, a white layer formation was found on the
top surface of the treated blood plasma samples and at the interfacing edge with a coppersurface, whereas the blood plasma without DBD treatment did not exhibit any whiteparticle formation on the sample surface after being exposed to open atmosphere for thesame duration (see Fig. The viscosity of blood plasma treated with DBD measured withBrookfield viscometer at two different shear rates, 225 and 450 s-1, is shown in Fig. Theviscosity of blood plasma showed irregular values in accordance with the DBD treatmenttime for both shear rates.
The values of WBV for the blood samples mixed with blood plasma treated by DBD are
given in Fig. at five different shear rates at each treatment time (i.e., 1, 2, 4, and 8 min).
Over the range of shear rates, the normalized WBV increased with increasing DBDtreatment time. WBV measured at a high shear rate of 300 s-1 is usually termed as systolicwhole blood viscosity (SBV), whereas WBV measured at a low shear rate of 1 s-1 istermed as diastolic whole blood viscosity (DBV) as WBV varies during a cardiac cycle likeblood pressure. As shown in Fig. DBV values were significantly higher in DBD-treatedsamples (i.e. 368.10 ± 65.41 mP for 8-min DBD treatment case) than untreated controlsamples (301.02 ± 56.17 mP). SBV values were also elevated from 38.60 ± 3.39 mP forcontrol to 49.30 ± 9.92 mP (for 8-min DBD treatment case).
WBVs of blood samples remixed with DBD-treated blood plasma, which was filtered before
being mixed with the original red blood cells are shown in Figs. and For SBV values shownin Fig. , the systolic blood viscosity after 8-min DBD treatment without filtration increased by5.5% from the baseline value of 41.0 mP. When the DBD-treated plasma was filtered and thenremixed with red blood cells, the systolic blood viscosity decreased by 9.1% from the baselineSBV of 41.0 mP. The diastolic blood viscosity of blood treated by DBD but without filtrationwas elevated with increasing DBD treatment time. For example, the DBV of blood increased by29.9% from the baseline value of 340.7 mP (see Fig. ). When the DBD-treated plasma wasfiltered then remixed with red blood cells, the DBV of blood for 8-min treatment case decreasedby 17.7% from the baseline value of 340.7 mP.
When blood plasma was treated by DBD, a white clot layer was formed at the top of bloodplasma sample (see Fig. suggesting that the plasma proteins and lipids in blood plasmamight have precipitated into coagulated particles through DBD treatment. Accordingly, if
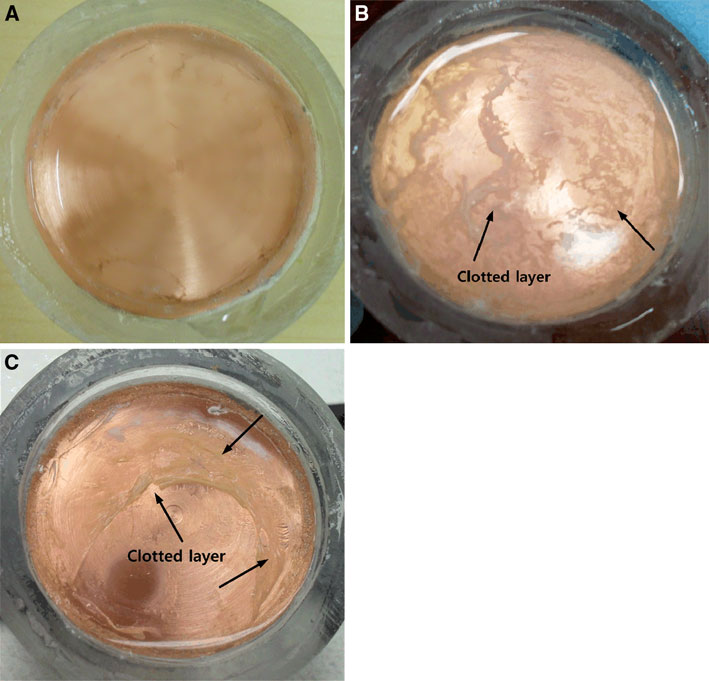
Plasma Chem Plasma Process (2012) 32:165–176
Fig. 3 Photographs of the formation of white layer in blood plasma sample with DBD treatment: a bloodplasma before DBD treatment showed no coagulation, b blood plasma treated with DBD for 4 min.
exhibited a partially coagulated layer, and c blood plasma treated with DBD for 8 min. Showed white clottedlayer on the surface of copper surface
these coagulated particles are removed, one may anticipate some improvement in therheological properties of whole blood. Note that the size of fibrinogen (diameter of 5–7 nmand length of 48 nm) and LDL molecules (approximately 22 nm) are too small to beremoved by filter [].
Although DBD discharge employed in this study is non-thermal, there could be about
20% of thermal energy to be transferred from filamentary discharge to the blood plasmasample due to energy distribution of input power to the metallic electrode [aphenomenon which could create the white layer of coagulated particles by heating effect.
To test this possibility, temperature measurement was performed before and after thetreatment of the samples with DBD, and no significant change in temperature wasobserved, suggesting that the coagulated particles must have come from the oxidation andsubsequent coagulation by active species generated by DBD. In addition, there was nochange in hematocrit between before and after DBD treatment, indicating no significantevaporation in blood plasma during the DBD treatment procedure.
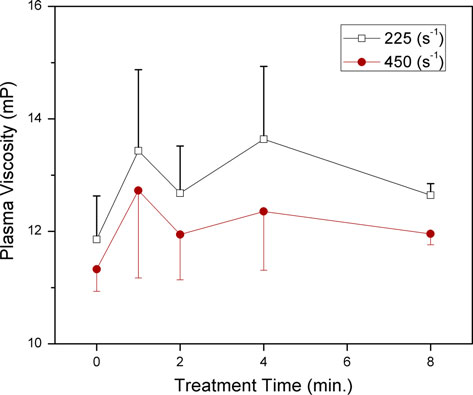
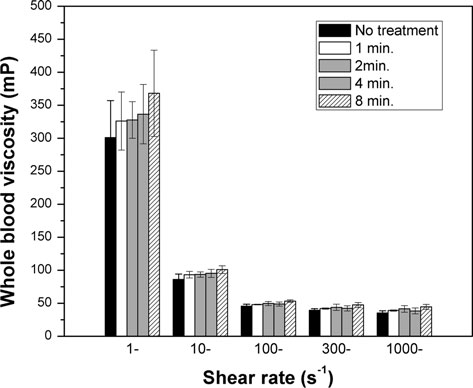
Plasma Chem Plasma Process (2012) 32:165–176
Fig. 4 Variations in the viscosity of blood plasma at two different shear rates, 225 and 450 s-1 at fourdifferent DBD treatment times together with baseline data
Fig. 5 Variations in the viscosity of whole blood at four different DBD treatment times together withbaseline data
Two important buffer systems exist in blood plasma, dihydrogen phosphate buffer and
carbonic acid buffer. The dihydrogen phosphate buffer resists a drop in pH, while thecarbonic acid buffer resists a rise in pH []. It has been known that when non-thermalplasma discharge is applied to liquids, it generates a siginificant amount of hydrogen ions,which can change the acidity of liquids. The effect of DBD discharge on phosphatebuffered liquids which is similar to the buffering system of blood plsama has beeninvestigated and confirmed that when about 5-mL phosphate buffered solution was treatedwith DBD discharge, the phosphate buffer system prevented the acidification of the liquids,
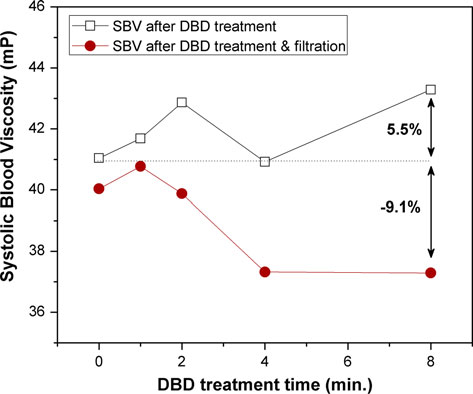
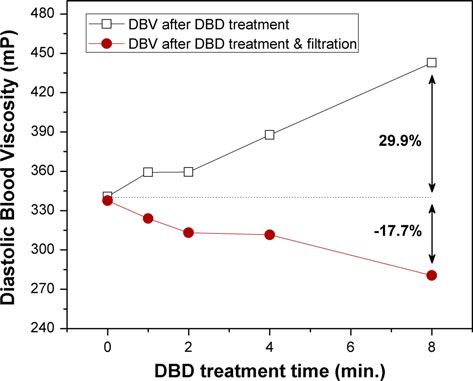
Plasma Chem Plasma Process (2012) 32:165–176
Fig. 6 Variations in the systolic blood viscosity of whole blood after DBD treatment with and withoutfiltration
Fig. 7 Variations in the diastolic blood viscosity of whole blood after DBD treatment with and withoutfiltration
while treating smaller volume (i.e. less than about 1.5 mL) for longer than about 10 min.
could be susceptible to the acidification of DBD discharge []. In the present study,the 4-mL blood plasma sample was treated with DBD discharge for maximum 8 min., thuswe assumed that the acidification of 4-mL blood plasma sample could not be significantlyaffected by DBD plasma discharge.
The blood mixed with the blood plasma treated by DBD without filtration showed
significant increase in WBV. This can be attributed to the fact that plasma proteins andlipids formed large groups of coagulated particles of micron size, increasing frictional
Plasma Chem Plasma Process (2012) 32:165–176
resistance to flow over the entire range of shear rates. Since the coagulated particles arevisible (i.e., large in size), they could be relatively easily removed with filtration, a processwhich helped reducing WBV as the plasma proteins and lipids are the key determinants ofWBV.
The SBV of blood with DBD treatment and filtration decreased by about 9.1%, whereas
the DBV dropped by 17.7% from the respective baseline values, both representing sig-nificant improvements in rheological properties. The reason why the DBD treatmentimproved the DBV more than the SBV is as follows: The SBV is usually affected byerythrocyte deformability while DBV is affected by the erythrocyte aggregation. Since theplasma proteins (i.e., fibrinogen and immunoglobulins) and LDL molecules are instru-mental in the RBC aggregation [, their removal by the DBD treatment and filtrationshould mitigate the erythrocyte aggregation, subsequently reducing the DBV.
In terms of the mechanism of DBD treatment in the precipitation of plasma proteins and
lipids from blood plasma, Kalghatgi et al. [reported that DBD treatment might haveactivated some of protein coagulation process which resulted in rapid fibrinogen aggregation.
It was also mentioned that the selective coagulation of proteins was observed—not ofalbumin but of fibrinogen only []. We can hypothesize that this coagulated fibrinogen andsubsequent removal might have affected the WBV of blood with DBD-treated blood plasma.
In addition, oxidized LDL molecules are known adhere to arterial wall surfaces, playing
a key role in the progression of atherosclerosis , In a similar manner, from thereduction of DBV, we can indirectly hypothesize that LDL molecules in blood plasmamight have been oxidized by DBD treatment, and the oxidized LDL molecules may tend toprecipitate and coagulate, a phenomenon which requires further investigation. Since LDLmolecules are one of the important variables in WBV [], their removal with DBDtreatment and filtration should not only help reducing WBV [but also reducing theprogression of atherosclerosis.
The present study utilized DBD treatment and filtration to reduce blood viscosity. Theresults presented in this paper indicated that the non-thermal DBD treatment could pre-cipitate and coagulate plasma proteins (i.e., fibrinogen) and LDL molecules in bloodplasma. The formation of white layer on the surface of blood plasma after DBD treatmentconfirmed the precipitation and coagulation of plasma proteins and lipids in blood plasma.
The present study showed that WBV could be significantly reduced by 9.1 and 17.7% forSBV and DBV, respectively, from the respective baseline values when DBD-treated bloodplasma was filtered prior to mixing. Further investigation is necessary to determine themechanisms of the precipitation and coagulation of plasma proteins and lipids by DBDnon-thermal plasma treatment.
1. Lee AJ, Mowbray PI, Lowe G, Rumley A, Fowkes FGR, Allan PL (1998) Blood viscosity and elevated
carotid intima-media thickness in men and women: the Edinburgh Artery Study. Circulation97(15):1467
2. Caimi G, Valenti A, Lo Presti R (2007) Acute myocardial infarction in young adults: evaluation of the
haemorheological pattern at the initial stage, after 3 and 12 months. Ann Ist Super Sanita 43(2):139–143
Plasma Chem Plasma Process (2012) 32:165–176
3. Ciuffetti G, Schillaci G, Lombardini R, Pirro M, Vaudo G, Mannarino E (2005) Prognostic impact of
low-shear whole blood viscosity in hypertensive men. Eur J Clin Invest 35(2):93–98
4. Lee BK, Durairaj A, Mehra A, Wenby RB, Meiselman HJ, Alexy T (2008) Hemorheological abnor-
malities in stable angina and acute coronary syndromes. Clin Hemorheol Microcirc 39(1–4):43–51
5. Chien S (1986) Blood rheology in myocardial infarction and hypertension. Biorheology 23(6):633–6536. Ernst E, Matrai A, Marshall M (1988) Blood rheology in patients with transient ischemic attacks. Stroke
7. Fisher M, Meiselman HJ (1991) Hemorheological factors in cerebral ischemia. Stroke 22(9):1164–11698. Velcheva I, Antonova N, Titianova E, Damianov P, Dimitrov N, Dimitrova V (2008) Hemorheological
disturbances in cerebrovascular diseases. Clin Hemorheol Microcirc 39(1–4):391–396
9. Tsuda Y, Satoh K, Kitadai M, Takahashi T (1997) Hemorheologic profiles of plasma fibrinogen and
blood viscosity from silent to acute and chronic cerebral infarctions. J Neurol Sci 147(1):49–54
10. Cecchi E, Marcucci R, Poli D, Antonucci E, Abbate R, Gensini GF, Prisco D, Mannini L (2006)
Hyperviscosity as a possible risk factor for cerebral ischemic complications in atrial fibrillation patients.
Am J Cardiol 97(12):1745–1748
11. Coull BM, Beamer N, de Garmo P, Sexton G, Nordt F, Knox R, Seaman GV (1991) Chronic blood
hyperviscosity in subjects with acute stroke, transient ischemic attack, and risk factors for stroke. Stroke22(2):162–168
12. Le Devehat C, Khodabandehlou T, Vimeux M (2001) Impaired hemorheological properties in diabetic
patients with lower limb arterial ischaemia. Clin Hemorheol Microcirc 25(2):43–48
13. Di Perri T, Forconi S, Agnusdei D, Guerrini M, Laghi Pasini F (1978) The effects of intravenous
isoxsuprine on blood viscosity in patients with occlusive peripheral arterial disease. Br J Clin Pharmacol5(3):255–260
14. Smith FB, Lee AJ, Hau CM, Rumley A, Lowe GD, Fowkes FG (2000) Plasma fibrinogen, haemostatic
factors and prediction of peripheral arterial disease in the Edinburgh Artery Study. Blood CoagulFibrinolysis 11(1):43–50
15. Ando J, Yamamoto K (2009) Vascular mechanobiology: endothelial cell responses to fluid shear stress.
Circ J 73(11):1983–1992
16. Libby P, Okamoto Y, Rocha VZ, Folco E (2010) Inflammation in atherosclerosis: transition from theory
to practice. Circ J 74(2):213–220
17. Caro CG (2009) Discovery of the role of wall shear in atherosclerosis. Arterioscler Thromb Vasc Biol
18. Falk E (2006) Pathogenesis of atherosclerosis. J Am Coll Cardiol 47(8):C7–C1219. Malek AM, Alper SL, Izumo S (1999) Hemodynamic shear stress and its role in atherosclerosis. JAMA
20. Merrill EW, Cokelet GC, Britten A, Wells RE Jr (1963) Non-Newtonian rheology of human blood:
effect of fibrinogen deduced by ‘‘subtraction''. Circ Res 13:48–55
21. Baskurt OK, Meiselman HJ (2003) Blood rheology and hemodynamics. Semin Thromb Hemost
22. Stoltz JF, Singh M, Riha P (1999) Hemorheology in practice. IOS Press, Wahington, DC23. Baskurt OK, Meiselman HJ (2007) Hemodynamic effects of red blood cell aggregation. Indian J Exp
Biol 45(1):25–31
24. Bandello F, Vigano D'Angelo S, Parlavecchia M, Tavola A, Della Valle P, Brancato R, D'Angelo A
(1994) Hypercoagulability and high lipoprotein(a) levels in patients with central retinal vein occlusion.
Thromb Haemost 72(1):39–43
25. Caen JP, Soria J, Collet JP, Soria C (1993) Fibrinogen, a vascular risk factor. Bull Acad Natl Med 177
(8):1433–1441; discussion 1441–1434
26. Chien S, Usami S, Dellenback RJ, Gregersen MI, Nanninga LB, Guest MM (1967) Blood viscosity:
influence of erythrocyte aggregation. Science 157(3790):829–831
27. Baskurt OK, Meiselman HJ (2009) Red blood cell ‘‘aggregability''. Clin Hemorheol Microcirc
28. Pasternak RC, Smith SC Jr, Bairey-Merz CN, Grundy SM, Cleeman JI, Lenfant C (2002) ACC/AHA/
NHLBI clinical advisory on the use and safety of statins. Circulation 106(8):1024
29. Amarenco P, Bogousslavsky J, Callahan A III, Goldstein LB, Hennerici M, Rudolph AE, Sillesen H,
Simunovic L, Szarek M, Welch KM, Zivin JA (2006) High-dose atorvastatin after stroke or transientischemic attack. N Engl J Med 355(6):549–559
30. Davignon J (2001) Advances in lipid-lowering therapy in atherosclerosis. Adv Exp Med Biol 498:49–5831. LaRosa JC, He J, Vupputuri S (1999) Effect of statins on risk of coronary disease. JAMA 282(24):234032. Baigent C, Keech A, Kearney PM, Blackwell L, Buck G, Pollicino C, Kirby A, Sourjina T, Peto R,
Collins R, Simes R (2005) Efficacy and safety of cholesterol-lowering treatment: prospective
Plasma Chem Plasma Process (2012) 32:165–176
meta-analysis of data from 90, 056 participants in 14 randomised trials of statins. Lancet 366(9493):1267–1278
33. Collins R, Armitage J, Parish S, Sleight P, Peto R (2004) Effects of cholesterol-lowering with sim-
vastatin on stroke and other major vascular events in 20536 people with cerebrovascular disease or otherhigh-risk conditions. Lancet 363(9411):757–767
34. Kearney PM, Blackwell L, Collins R, Keech A, Simes J, Peto R, Armitage J, Baigent C (2008) Efficacy
of cholesterol-lowering therapy in 18, 686 people with diabetes in 14 randomised trials of statins: ameta-analysis. Lancet 371(9607):117–125
35. Banyai S, Banyai M, Falger J, Jansen M, Alt E, Derfler K, Koppensteiner R (2001) Atorvastatin
improves blood rheology in patients with familial hypercholesterolemia (FH) on long-term LDLapheresis treatment. Atherosclerosis 159(2):513–519
36. Antons KA, Williams CD, Baker SK, Phillips PS (2006) Clinical perspectives of statin-induced
rhabdomyolysis. Am J Med 119(5):400–409
37. Kiortsis D, Filippatos T, Mikhailidis D, Elisaf M, Liberopoulos E (2007) Statin-associated adverse
effects beyond muscle and liver toxicity. Atherosclerosis 195(1):7–16
38. Cohen DE, Anania FA, Chalasani N (2006) An assessment of statin safety by hepatologists. Am J
Cardiol 97(8):S77–S81
39. Kalghatgi SU, Fridman G, Cooper M, Nagaraj G, Peddinghaus M, Balasubramanian M, Vasilets VN,
Gutsol AF, Fridman A, Friedman G (2007) Mechanism of blood coagulation by nonthermal atmo-spheric pressure dielectric barrier discharge plasma. IEEE Trans Plasma Sci 35(5):1559–1566
40. Fridman G, Shereshevsky A, Jost MM, Brooks AD, Fridman A, Gutsol A, Vasilets V, Friedman G
(2007) Floating electrode dielectric barrier discharge plasma in air promoting apoptotic behavior inmelanoma skin cancer cell lines. Plasma Chem Plasma Process 27(2):163–176
41. Stoffels E, Kieft I, Sladek R (2003) Superficial treatment of mammalian cells using plasma needle.
J Phys D Appl Phys 36:2908
42. Stoffels E, Sladek R, Kieft I (2004) Gas plasma effects on living cells. Physica Scripta 2004:7943. Fridman G, Peddinghaus M, Balasubramanian M, Ayan H, Fridman A, Gutsol A, Brooks A (2006)
Blood coagulation and living tissue sterilization by floating-electrode dielectric barrier discharge in air.
Plasma Chem Plasma Process 26(4):425–442
44. Fridman G, Friedman G, Gutsol A, Shekhter AB, Vasilets VN, Fridman A (2008) Applied plasma
medicine. Plasma Process Polym 5(6):503–533
45. Chirokov A, Gutsol A, Fridman A (2005) Atmospheric pressure plasma of dielectric barrier discharges.
Pure Appl Chem 77(2):487–495
46. Kalghatgi SU, Fridman G, Fridman A, Friedman G, Clyne AM (2008) Non-thermal dielectric barrier
discharge plasma treatment of endothelial cells. In 2008. IEEE, pp 3578–3581
47. Dobrynin D, Fridman G, Friedman G, Fridman A (2009) Physical and biological mechanisms of direct
plasma interaction with living tissue. New J Phys 11:115020
48. Ayan H, Fridman G, Staack D, Gutsol AF, Vasilets VN, Fridman AA, Friedman G (2009) Heating
effect of dielectric barrier discharges for direct medical treatment. IEEE Trans Plasma Sci37(1):113–120
49. Kim S, Cho YI, Hogenauer WN, Kensey KR (2002) A method of isolating surface tension and yield
stress effects in a U-shaped scanning capillary-tube viscometer using a Casson model. J Non-NewtonFluid Mech 103(2–3):205–219
50. Matrai A, Whittington R, Ernst E (1985) Correction of blood viscosities to standard haematocrit: a
simple new method. Clin Hemorheol 5:622
51. Matrai A, Whittington R, Ernst E (1987) A simple method of estimating whole blood viscosity at
standardized hematocrit. Clin Hemorheol 7:261–265
52. Hall CE, Slayter HS (1959) The fibrinogen molecule: its size, shape, and mode of polymerization.
J Biophys Biochem Cytol 5(1):11
53. Mora S, Szklo M, Otvos JD, Greenland P, Psaty BM, Goff DC (2007) LDL particle subclasses, LDL
particle size, and carotid atherosclerosis in the Multi-Ethnic Study of Atherosclerosis (MESA). Ath-erosclerosis 192(1):211–217
54. El Harchaoui K, van der Steeg WA, Stroes ESG, Kuivenhoven JA, Otvos JD, Wareham NJ, Hutten BA,
Kastelein JJP, Khaw KT, Boekholdt SM (2007) Value of low-density lipoprotein particle number andsize as predictors of coronary artery disease in apparently healthy men and women: The EPIC-norfolkprospective population study. J Am Coll Cardiol 49(5):547–553
55. Jidenko N, Bourgeois E, Borra J-P (2010) Temperature profiles in filamentary dielectric barrier
discharges at atmospheric pressure. J Phys D Appl Phys 43(29):295203
56. Nozaki T, Miyazaki Y, Unno Y, Okazaki K (2001) Energy distribution and heat transfer mechanisms in
atmospheric pressure non-equilibrium plasmas. J Phys D Appl Phys 34:3383–3390
Plasma Chem Plasma Process (2012) 32:165–176
57. Bray JJ (1999) Lecture notes on human physiology, 2nd edn. Wiley, Blackwell58. Oehmigen K, Ha¨hnel M, Brandenburg R, Wilke C, Weltmann KD, von Woedtke T (2010) The role of
acidification for antimicrobial activity of atmospheric pressure plasma in liquids. Plasma Process Polym7(3–4):250–257
59. Libby P, Ridker PM, Maseri A (2002) Inflammation and atherosclerosis. Circulation 105(9):1135–114360. Epstein FH, Fuster V, Badimon L, Badimon JJ, Chesebro JH (1992) The pathogenesis of coronary
artery disease and the acute coronary syndromes. N Engl J Med 326(4):242–250
61. Sloop GD, Garber DW (1997) The effects of low-density lipoprotein and high-density lipoprotein on
blood viscosity correlate with their association with risk of atherosclerosis in humans. Clin Sci (Lond)92(5):473–479
62. Sloop GD, Mercante DE (1998) Opposite effects of low-density and high-density lipoprotein on blood
viscosity in fasting subjects. Clin Hemorheol Microcirc 19(3):197–203
63. Slyper A, Le A, Jurva J, Gutterman D (2005) The influence of lipoproteins on whole-blood viscosity at
multiple shear rates. Metabolism 54(6):764–768
64. Moriarty PM, Gibson CA, Kensey KR, Hogenauer W (2004) Effect of low-density lipoprotein
cholesterol apheresis on blood viscosity. Am J Cardiol 93(8):1044–1046
Source: http://gregfridman.org/support/pdf/2012-PCPP-blood.pdf
MISE EN FORME ET CORRECTION : Dr Claudine RESSEL-SCHUMACHER
Avec la col aboration de : Dr Roselyne ILTIS et Isabel e WENGER, IDE Groupe Expert de la Société de Gérontologie de l'Est
Dr Claire GROSSHANS
Pôle de Gérontologie Clinique MULHOUSE
Merci à AGIRA pour son soutien financier 1. Généralités……………………………………………… p. 1 à 24 2. Rétention urinaire – Dysurie …………………… p. 25 à 32 3. Instabilité vésicale – Hyperactivité vésicale . p. 33 à 41 4. Polyurie nocturne et Nycturie …………………. p. 42 à 52 5. Infection urinaire …………………………………… p. 53 à 58 6. Rééducation sphinctérienne ……………………. p. 59 à 67 7. Traitements médicamenteux …………………. p. 68 à 97 8. Sondage vésical ……………………………………. p. 98 à 102 9. Incontinence fécale – Constipation …………. p. 103 à 106 10. Divers ………………………………………………… p. 107 à 120 Auteurs Lexique
FEI Check List - Draft Schedule DENOMINATION OF EVENT DATE (dd/mm/yy): 18/02/2013 – 20/02/2013PLACE: Vestfold, Oslofjord Convention Center GENERAL CONDITIONS This event is organised in accordance with:- FEI Statutes, 23rd edition, effective 8th November 2012 FEI General Regulations, 23rd edition, effective 1st January 2009, updates effective FEI Veterinary Regulations, 13th edition, effective 1st January 2013