Microsoft word - lichtwirkungen_05_05_english_20110514_conni.doc
TU Ilmenau FG Lichttechnik Prof. Dr. sc. nat. Christoph Schierz Dr.-Ing. Cornelia Vandahl
Biological effects of light – Literature overview
1 Introduction
It has been known for considerable time that light does not only serve for obtaining visual
information but also has great influence on bodily functions. Many new aspects have been
established in recent years.
The non-visual information is received via light that falls onto our eyes and is conveyed via
a nerve connection (retinohypothalamic tract RHT) to the suprachiasmatic nucleus (SCN).
The SCN is the central circadian Zeitgeber for the day-night rhythm, for the control of the
body temperature and many other functions. Figure 1 shows the schematic coherence.
The non-visual system has its own receptors located in the retina. They are light sensitive
ganglion cells containing the photo pigment melanopsin [10], [25). They are called "intrin-
sically photosensitive retinal ganglion cells" (ipRGC). There number is about 1-3% of all
retinal ganglion cells. They also function as ganglion cells of the visual system at the same
time and therefore also receive signals from the rods and cones. In case of a failure of the
visual system they can continue to function independently [40].
The effect of light on the biological rhythm depends on the intensity of light, the duration of
exposure, the time regime, and the time of day, the spectral composition and the spatial
distribution of light. Effects have been verified even for low illuminance levels. Rea [47] has
demonstrated effects at 18 lx (blue LEDs). Zeitzer [61] has established effects at 106 lx
with polychromatic light from fluorescent lamps (4000K) and Smith [56] at 200 lx.
Figure 1: Simplified schematic of visual and non-visual effects of light [10]
2 Effects of light on the circadian rhythm
The bodily functions of people are subject to a circadian (approximate day periodic) rhyth-
micity. This rhythmicity is generated by the SCN (Nucleus Suprachiasmaticus) and is
about 24 hours [17]. The SCN acts as the inner clock controlling many physiological and
psychological functions [18]. This rhythm manifests itself in changes of the body core tem-
perature as well as the melatonin and cortisol levels. Furthermore it influences a multitude
of other bodily functions (Figure 2).
The sleep-wake pattern of human can clearly be recognised. The minimum body core
temperature is reached about 1 to 2 hours prior to awakening. The maximum of the mela-
tonin synthesis is in the middle of the sleeping phase, about 2 to 3 hours before the mini-
mum body core temperature (Figure 2) is reached. The beginning of the increase of mela-
tonin levels, in other words, the maximum melatonin secretion or the minimum body core
temperature are used for determining the individual phasing.
The phasing and the progression of the rhythmicity vary with the individuals. This results in
the so called chrono-types. The morning chrono-types experience an earlier melatonin
secretion compared to the evening chrono-types.
60
Plasma Melatonin [pg/ml]
Plasma Cortisol [μ
g/dl]
Core Body Temperature [°C]
Time of Day
Figure 2: Circadian rhythmiticy of cortisol, melatonin and body core temperature (as per Hofstra [32]).
Sleep regulation depends on the circadian rhythm (circadian component, C) and the sleep homeostatic component, S. The homeostatic sleep propensity increases during the alert-ness phase and is reduced while sleeping. The longer one is awake the greater it becomes. In the simplest case one can assume a linear progression [33]. Due to the increase of sleep propensity the homeostatic compo-nent of alertness rises during the day (Figure 3). The circadian alertness component increases after awakening and decreases again after about 10 to 12 hours. In a first approximation both components are additive. This assures that human can be awake during the whole day and subsequently enjoy a continuous sleep phase [8], [24],
[33], [36] (Figure 3). This cumulative curve of alertness must not be confused with the will-ingness to perform which varies with the time of day. More detailed models draw closer to the latter and take into account for instance the sleepiness after waking up [1] or the per-formance low experienced after noon [36].
Homeostatic (S) and
circadian (C) component
of alertness level. The total represents the
alertness. It is lowest at
night and thus facilitates a sleep phase lasting 8
hours (as per [8], [24], [33])
Since the duration of the circadian rhythm is not precisely 24 hours (in most cases it is somewhat longer than 24 hours) the inner clock must be synchronised by an external Zeit-geber. The decisive Zeitgeber is the bright-dark rhythm of daylight; other influencing fac-tors are, amongst others, social surroundings, temperature changes during the course of the day and food intake. The information regarding the availability of light is transported via special photo receptors in the eye to the inner, endogenous biological stimulator. This causes the suppression of melatonin secretion. Melatonin is transported via the blood circulation into all cells in the body and thus provides the information required for their synchronisation [18]. If the phas-ing of the inner clock does not coincide with the bright-dark phase of daylight (e. g: after intercontinental flights through several time zones) the synchronisation is also established via this mechanism. For the synchronisation due to light the time of exposure is decisive. Light in the evening causes a phase delay and therefore delays the rhythm, since the body receives signals indicating it was still day. Accordingly light in the early morning causes a phase advance. Light at noon only has a very limited influence on phase-shifting. Furthermore the intensity, the spectral composition as well as the distribution of light in terms of time and space play a role.
3 Activation due to light
The alertness level can be increased with light. The subjective fatigue decreases respec-
tively the subjective alertness increases with greater illuminance levels than 100 lx at the
cornea. Such activating effects due to light have been established objectively on physio-
logical parameters. The greatest effects can be achieved at 300 lx at the eye as per Ca-
jochen [13].
Thus light has the potential to activate and to increase performance independently from the phasing of the inner clock. However, in studies it is quite difficult to separate potential effects of light from other variable factors of motivation. Laboratory studies permit the con-clusion that high lighting levels do not only increase visual performance but generally im-prove work performance. This can be expressed by the reduced number of mistakes and an increased volume of work or better retentiveness. A comprehensive summary can be found at Cajochen [15].
Subjective Alertness
Cornea Illuminance [lx]
Subjective alertness depending on cornea illuminance (Cajochen [13]).
4 Spectral effect of monochromatic light (overview as per Brainard
Brainard names 8 action spectra in his publications [10] that were established in different research projects [3], [11], [27], [30], [31], [40]; [58], [61] on humans and animals using different methodologies. One example is the meanwhile well known curve as per Brainard [10] (Figure 5). All research was carried out using monochromatic light where the mela-tonin suppression was determined during the night. The results cannot be transferred to other times of the day or to polychromatic light by implication. However, they offer an ex-cellent base for the development of a model for melatonin suppressing effects of light.
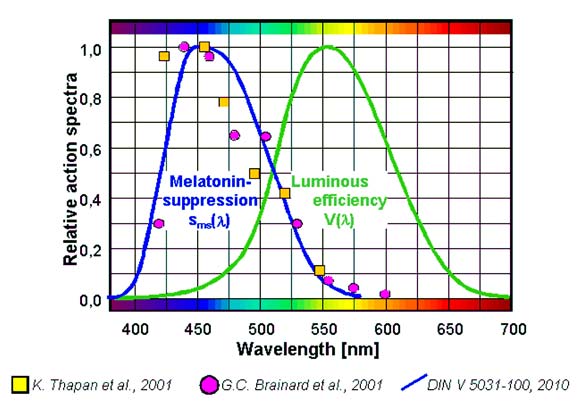
Figure 5: Action spectrum for melatonin suppression in humans at night (Brainard [10])
5 Spectral effect as per DIN V 5031-100
Since 2009 the action spectrum sms(λ) for nocturnal melatonin suppression is stipulated in
DIN V 5031-100 [19] (Figure 6). This is based on the same measurements as the curve in Figure 5. However, in the short wave range it is based on the transmission curve of the human eye [26].
Figure 6: Action spectrum for melatonin suppression in humans at night sms(λ) (DIN [19]
In DIN V 5031-100 the following quantities for the evaluation of the biological effects of light are defined:
Biological evaluation:
= X λ s
∫ ( )⋅ ( )⋅
∫ X (λ)⋅s (λ)⋅dλ
Biological action factor:
∫ X (λ)⋅V(λ)⋅dλ
Quantity derived from radiant quantity Xe by evaluating the
radiation according to its biological action
abiol v Biological action factor
λ λ ) Spectral distribution of the radiation
Action spectrum for biological effect,
related to maximum s
V (λ) Spectral luminous efficiency for photopic vision
Limiting wavelength
The defined spectral sensitivity sms(λ) was used for the evaluation of the melatonin sup-
X (λ) s (λ) d
Besides the melatonin suppression light can also delay the circadian rhythm, can change the circadian amplitude and affect activation. Currently there are no exact findings regard-ing the action spectrum of these effects. There is, however, research [14], [38], that implies the action spectra are similar to those for the melatonin suppression. Therefore it is cur-rently suggested to use this action spectrum for all effects mentioned. Differing from this it is recommended to employ the V(λ) curve for the evaluation of effects of light in conjunction with the treatment of seasonally acquired depression (SAD). How-ever, there is no scientific basis for this.
6 Spectral effect of polychromatic light
Figueiro [22], [23] and Rea [47] report of research where a comparison of the melatonin
suppressing effects of two different types of radiation (LED and high pressure mercury va-
pour lamp) was carried out. The cornea illuminance generated by the LEDs was 18 lx that
by the mercury vapour lamp was 450 lx. The effective radiation level (weighted as per the
curve in Figure 5) was about twice as high for the mercury vapour lamp while the mela-
tonin suppression was only half. According to Figueiro the yellow spectral components
reduce the effectiveness of short wave light for the melatonin production at night; the cir-
cadian system does not work additively. Under certain circumstances the total effect may
be less than the effect of the individual components. This effect is taken into account in a
proposed effectiveness curve (Figure 7) which includes negative values that take into ac-
count the assumed compensatory effect of long wave radiation on the melatonin suppres-
sion.
Figure 7: Action spectrum for melatonin suppression in humans for polychromatic light (Figueiro [22])
In a further study by Rea [48] 4 white light sources (fluorescent lamps) with different corre-lated colour temperatures (CCT) were compared with each other. The suppressive effect on the concentration of melatonin in the blood was determined for two illuminance levels (300 lx and 600 lx) for each lamp type after one hour radiation at night. A higher level of melatonin suppression was found with all lamp types at the higher illuminance level. It was also determined that the CCT is not a reliable measure for describing the efficiency of the melatonin suppression. Lamps with lower CCT can facilitate the same or even higher me-latonin suppression at night than lamps with a high correlated colour temperature at the same lighting level (Figure 8).
Figure 8: Melatonin suppression for various colour temperatures (300 . 600 lx) [48]
7 Dependency of the effect on the location of the illumination on the
More recent studies came to the conclusion that the different areas of the retina of the hu-man eye respond with different sensitivity to light. From a research project [28] it can be deducted that light from above is more effective for the melatonin suppression than light from below (Figure 9). Lasko [37] also published similar results. According to Rüger [52] lighting from the nose side is more effective than from the tem-ples. This effect is less important since it is compensated for two-eye vision. Two eyes are more effective for the melatonin suppression than one on its own [59].
Figure 9: Melatonin suppression subject to the
size of the area [28]
200 lx Lower 200 lx Upper
Full: whole retina
Retinal Exposure Conditions
Lower: lower retinal area Upper: upper retinal area
elatonin pg/ml (MW+-SEM)M
Melatonin suppression subject to the
retinal area [52]
Dim: no lighting
Nasal: lighting from the nose side Temporal: lighting form the temples
8 Time dependency of the effect
Studies indicate that the sensitivity to light can vary over time. Figueiro describes a study
[23] in which the melatonin suppression was compared for two light scenes (lighting with
mercury vapour lamp at 450 lx and LED s at 18 lx) in two test periods of 1 hour each after
midnight. It was established that the melatonin suppression was greater with both light
sources during the second test compared to the first one (Figure 11).
Figure 11: Average melatonin suppression for two test periods of 1 hour each in the same night employing mercury vapour lamps and LEDs [23]
Another study by Smith [56] has also shown that the light scenes prior to the test have an influence on the effect of light. Figure 12 shows three different test periods of the light scenes. The cases B and C show exposure to 200 lx over 6.5 hours after midnight. The light scene prior to this test was significantly different (200 lx vs. 0.5 lx generated by fluo-rescent lamps with 4100 K in both cases). The influence of the progression over time on the melatonin content can be clearly seen. Similar or equal illuminance levels before and during the test (case A and B) show less impact than a considerable change of the lighting level.
Melatonin secretion for 3 different lihgting scenes
Start ca. 00:00 a.m.
over time, the graph shows the individual profiles for each of the 8 test persons [56]
Some studies [2], [7], [12], [29], [50] compare continuous lighting over time with a scenario of short times of exposure to very bright light. In all these studies high lighting levels were applied which allows the assumption that a saturation effect has already set in. 10 to 15 minutes bright light interrupted by 30 to 80 minutes of darkness practically have the same effect as continuous lighting with the same illuminance level (Figure 13). A summary and modelling can be found at Kronauer [35].
Time Relative to Baseline DLMO (h)
Time Relative to Baseline DLMO (h)
Time Relative to Baseline DLMO (h)
Figure 13: Phase advance with 3.5 h light exposure each in the morning [12]; Dim: <60 lx, Intermittent: ca. 6000 lx / 60 lx alternately every 0.5 h, Continuous: ca. 6000 lx (with fluroescent lamps 4100 K), there is no significant variation betweeen intermittent and continuous illumination (DLMO (Dark Light Melatonin Onset) Start of the melatonin secretion without lighting effect).
The intermittent lighting regime represents a good alternative for workplaces that cannot be illuminated continuously with sufficient lighting levels. For instance one could light the break room or cafeteria with such lighting and assure that workers get enough bright light by managing the schedule of coffee breaks.
9 Light and Dementia
A study by the TU Ilmenau [4], [5] researched the effect of light on people suffering from
dementia. In a nursing home for the elderly a luminous ceiling was installed in the lounge
area where lighting parameters such as illuminance level and colour temperature could be
adjusted.
During a test series in February/March 2005 the light scenes were altered in phases in
order to investigate the influence of the quality of light on the residents. During these tests
the lighting level as well as the effective radiation intensity was increased considerably in
phase II compared to phase I. The analysis has shown that in phase II with high lighting
levels and high biological action spectra in the lounge area the test persons had less sleep
phases during the day and enjoyed better sleep quality during the night. Furthermore it
was established that even though there was no general improvement of the basic illness, a
certain higher level of activity was noted which manifested itself by a better ability of orien-
tation and higher emotional stability in phase II (Figure 14). Thus the quality of life of the
people concerned can be improved due to increased initiative of the residents, better ori-
entation and enhanced well-being.
Reports by other authors at the CIE convention 2006 in Ottawa [16] have shown similar
results.
Mean value and standard deviation of the group for each phase
(6 persons)
occurence 2
parameter / phase
Figure 14: Change of behaviour of the test persons in phase II compared to phase I (significant changes are marked with *) [5]
10 Risk of cancer
There is a number of studies that indicate that exposure to light during the night can abet
the development of cancer. However, there are also studies claiming the opposite.
About 30 global studies regarding the relationship between shift work and cancer have
been evaluated at the Institute for Occupational Health, Social Medicine and Public Health
at the University of Cologne [21]. One key result of these analyses is that in both re-
searched groups of test persons – namely flight personnel and shift workers – a statisti-
cally significant increase of the risk of getting cancer was established. The risk increases
with the number of years a person works shifts. An increased risk of about 40 – 70% com-
pared to the non-shift working population is given.
Light could be one possible cause for this accumulation of cancer for shift workers. At
night light prevents the production of the hormone melatonin which has been proven to
initiate regeneration and repair processes. Melatonin also protects the cells from potential
damage due to free radicals. Erren [20] provided an overview over these studies.
It is assumed that melatonin has an inhibiting effect against cancer [6], [55]. Research re-
garding shift work and sleeping behaviour of cancer patients also indicates an increased
risk of cancer due to nightly exposure to light. Furthermore it is known that melatonin inhib-
its the production of estrogens. The estrogenic level increases if less melatonin is pro-
duced. Estrogens play an important role in the development of breast cancer. Here there is
also an indication of a link to exposure to light at night.
In October 2007 the International Agency for Cancer Research (IARC), a facility of the
World Health Organisation, has classified shift work at night as probably cancer-causing.
In the explanatory statement is says: "Epidemiological studies have found that long-term
night workers have a higher risk of breast cancer than women who do not work at night. …
The studies are consistent with animal studies that demonstrate that constant light, dim
light at night, or simulated chronic jet lag can substantially increase tumour development.
Other experimental studies show that reducing melatonin levels at night increases the in-
cidence or growth of tumours." [34].
A further summary can be found at Megdal [42], Pauley [45] and Stevens [57].
11 Summary
The following statements can be deducted from the given overview and in support of
Boyce [9]:
1. It is known that light has an effect on the melatonin suppression but it does provide an
explanation of the effects on the circadian processes. Anatomic studies have shown that the SCN is connected to many other parts of the brain such as the hypothalamus, the pituitary gland, the septum and the mesencephalon (midbrain). These parts regu-late the production of a wide range of hormones which probably have an impact on many different physiological functions.
2. The spectral effect of polychromatic light (and thus for almost all relevant lighting ap-
plications) has only been researched in a small number of studies and thus is not yet securely defined.
sms(λ) curve only describes the melatonin suppressive effect of monochromatic
light and thus only part of the biological effects of light.
4. There are research studies that show that other parameters also have an influence on
the melatonin suppressing effect such as:
- The local distribution on the retina - The point in time of exposure to light - The lighting scenarios of the previous hours and days (regarding lighting level,
spectrum and time duration)
All these parameters must be implemented into the action spectrum in order to be able to predict the effect.
5. It is also important to look for the negative effects of light on the circadian rhythm. The
fact that there may well be negative effects is indicated by the relationship between light therapy and in case of SAD ailments and the accelerated growth of tumours in case of exposure to light during the night. These findings should serve as a warning against the hasty application of light as a measure for improving one's health.
6. The current level of knowledge is not sufficient for the deduction and development of
control concepts for the lighting of work places. There are still many questions to be answered if light is to be used for stimulating the circadian system. Currently the spec-tral sensitivity, the relative spectral sensitivity of different retina zones, the question if adaptation processes exist as in the visual system, how the exposure to light over time can be integrated and the influence of the control over time of the exposure to light are of primary importance.
12 Literature overview
[1] Achermann, P., Borbély, A.A. (1994): Simulation of daytime vigilance by the additive
interaction of a homeostatic and a circadian process. Biol. Cybern. Vol. 71, Nr. 2, S. 115-121.
[2] Baehr, E.K., Fogg, L.F., Eastman, C.I. (1999): Intermittent bright light and exercise to
entrain human circadian rhythms to night work. American Journal of Physiology-Regulatory Integrative and Comparative Physiology, Vol. 277 Nr. 6 S. R1598-R1604
[3] Berson, D. M.; Dunn, F. A.; Takao, M.: Phototransduction by retinal ganglion cells that
set the circadian clock, Science 295, pp. 1070-1073, 2002
[4] Bieske, K:; Dierbach, O.: Evaluation des Einsatzes von tageslichtähnlichem Kunstlicht
in der gerontopsychiatrischen Pflege und Betreuung Hochbetagter, Tagung Licht und Gesundheit Berlin 2006
[5] Bieske, K.; Gall, D.; Vandahl, C.; Dierbach, O.: Influence of artifical light on geron-
topsychiatric care of elderly people, Proc. Symp. „Lighting & Health" CIE x032:2006, pp.106-109
[6] Blask, D. E.; Brainard, G. C.; Dauchy, R. T.; Hanifin, J. P.; Davidson, L. K.; Krause, J.
A.; Lynch, D. T.; Sauer, L. A., Jasser, S. A.: Melatonin Suppression by Ocular Light Exposure During Darkness: Impact on Cancer Growth and Implications for Cancer Risk in Humans, Proc. Symp. 2004 „Light & Health" CIE x027: 2004, pp. 42-45, 2004
[7] Boivin, D.B., James, F.O. (2005): Light treatment and circadian adaptation to shift
work. Industrial Health, Vol. 43 Nr. 1 S. 34-48
[8] Borbely, A. A., Acherman, P., Trachsel, L. and Tobler, I. (1989): Sleep initiation and
initial sleep intensity: interaction of homeostatic and circadian mechanisms. J. Biol. Rhythms Vol. 4 Nr. 2 S. 149-160.
[9] Boyce, P.: Implications for Lighting – What do we know? CIE Proc. Symp. „Lighting &
Health" Ottawa CIE x032:2006, pp. 27-31
[10] Brainard, G. C.; Hanifin, J. P.: The Effects of Llight on Human Health and Hehavior:
Relevance to Architectural Lighting, Proc. Symp. 2004 „Light & Health" CIE x027: 2004, pp. 2-16, 2004
[11] Brainard, G. C.; Hanifin, J. P.; Greeson, J. M.: Byrne, B.; Glickman, G.; Gerner, E.;
Rollag, M. D.: Action spectrum for melatonin regulation in humans: evidence for a novel circadian photoreceptor, J. Neurosci. 21, pp. 6405-6412, 2001
[12] Burgess, H. J.; Crowley, S. J.; Gazda, C. J.; Fogg, L. F.; Eastman, C. I.: Preflight Ad-
justment to Eastward Travel: 3 Days of Advancing Sleep with and without Morning Bright Light, Journal of Biological Rhythms Vol. 18 No. 4, August 2003, pp. 318-328
[13] Cajochen, C., Zeitzer, J. M., Czeisler, C. A., & Dijk, D.-J. (2000). Dose-response rela-
tionship for light intensity and ocular and electroencephalographic correlates of human alertness. Behavioural Brain Research 115 (1), S. 75-83
[14] Cajochen, C., Münch, M., Kobialka, S., Kräuchi, K., Steiner, R., Oelhafen, P., Orgül,
S., Wirz-Justice, A. (2005): High Sensitivity of Human Melatonin, Alertness, Thermo-regulation, and Heart Rate to Short Wavelength Light. J of Clinical Endocrinology & Metabolism Vol. 90, No. 3 S. 1311-1316
[15] Cajochen, C. (2007): Alerting effects of light. Sleep Medicine Reviews, Vol. 11 Nr. 6 S.
[16] CIE Proc. Symp. „Lighting & Health" Ottawa CIE x032: 2006 [17] Czeisler, C.A., Duffy, J.F., Shanahan, T.L., Brown, E.N., Mitchell, J.F., Rimmer, D.W.,
Ronda, J.M., Silva, E.J., Allan, J.S., Emens, J.S., Dijk, D.J., Kronauer, R.E. (1999): Stability, precision, and near 24-hour period of the human circadian pacemaker. Sci-ence Vol 284 S. 2177-2181.
[18] Dijk, D.J., Czeisler, C.A. (1995b): Contribution of the circadian pacemaker and the
Sleep Homeostat to Sleep Propensity, Sleep Structure, Electroencephalographic Slow Waves, and Sleep Spindle Activity in Humans. The Journal of Neuroscience, Vol. 15 Nr. 5 S. 3526-3538
[19] DIN V 5031-100: Strahlungsphysik im optischen Bereich und Lichttechnik – Teil 100 :
Über das Auge vermittelt, nichtvisuelle Wirkung des Lichts auf den Menschen – Größen, Formelzeichen und Wirkungsspektren
[20] Erren, T.C., Reiter, R.J., Piekarski, C. (2003): Light, timing of biological rhythms, and
chronodisruption in man. Naturwissenschaften, Vol. 90 Nr. 11 S. 485-494.
[21] Erren, T.C., Reiter, R.J., Piekarski, C. (2008): Chronodisruption and melatonin: the
need for sensible exposure metrics in epidemiological studies. Journal of Pineal Re-search, Vol. 45 Nr. 3 S. 335-336.
[22] Figueiro, M. G.; Bullough, J. D.; Parsons, R. H.; Rea, M.S.: Preliminary evidence for
spectral opponency in the suppression of melatonin by light in humans, Neuroreport 5, pp. 313-316, 2004
[23] Figueiro, M. G.; Bullough, J. D.; Parsons, R. H.; Rea, M.S.: Preliminary evidence for a
change in spectral sensitivity of the circadian system at night, Journal of circadian Rhythms 2005, 3:14
[24] Folkard, S., Akerstedt, T., Macdonald, I., Tucker, P., Spencer, M.B. (1999): Beyond
the three-process model of alertness: Estimating phase, time on shift, and successive night effects. Journal of Biological Rhythms, Vol. 14 Nr. 6 S. 577-587.
[25] Foster, R. G.: Bright Blue Times. Nature 433, pp. 698-699, 2005 [26] Gall, Dietrich (2002): Circadiane Lichtgrößen und deren messtechnische Ermittlung
Licht 54 (2002) 11/12, S. 1292–1297
[27] Gamlin, P. D.; Smith, V. C.; Dacey, D. M.; Pokorny, J.; McDougal, D. H.: Melanopsin-
containing retinal ganglion cells drive the pupillary light reflex in the primate, Invest. Ophthalmol. Vis. Sci. 45, pp. ARVO E-Abstract 2262, 2004
[28] Glickman, G.; Hanifin, J. P.; Rollag, M. D.; Wang, J.; Cooper, H.; Brainard, G. C.: Infe-
rior Retinal Light Exposure Is More Effective than Superior Retinal Exposure in Sup-pressing Melatonin in Humans, Journal of Biological Rhythms, Vol. 18 No. 1, pp 71-79, 2003
[29] Gronfier, C., Wright, K.P., Kronauer, R. E., Jewett, M.E., Czeisler, C.A. (2004): Effi-
cacy of a single sequence of intermittent bright light pulses for delaying circadian phase in humans. American Journal of Physiology-Endocrinology and Metabolism, Vol. 287 Nr. 1 S. E174-E181
[30] Hankins, M. W.; Lucas, R. J.: The primary visual pathway in humans is regulated ac-
cording to long-term light exposure through the action of a nonclassical photopigment, Current Biology 12, pp. 191-198, 2002
[31] Hattar, S., Lucas, R. J.; Mrosovsky, N.;, Thompson, S.; Douglas, R. H.; Hankins, M.
W.; Lem, J.; Biel, M.; Hofmann, F.; Foster, R. G.; Yau, K.-W.: Melanopsin and rod-cone photoreceptive systems account for all major accessory visual functions in mice, Nature 424, pp. 76-81, 2003
[32] Hofstra., W.A., de Weerd, Al W. (2008): How to assess circadian rhythm in humans: A
review of literature. Epilepsy & Behavior Vol. 13 Nr. 3 S. 438–444.
[33] Horowitz, T.S., Tanigawa, T. (2002): Circadian-based new technologies for night
workers. Industrial Health, Vol. 40 Nr. 3 S. 223-236.
[34] IARC (2007): IARC Monographs: http://www.iarc.fr/en/Media-Centre/IARC-Press-
[35] Kronauer; R.E., Forger, D.B., Jewett, M.E. (1999): Quantifying Human Circadian
Pacemaker Response to Brief, Extended, and Repeated Light Stimuli over the Pho-totopic Range. Journal of Biological Rhythms, Vol. 14 No. 6, S. 501-515
[36] Kunz, D. (2006): Melatonin und Schlaf-Wach-Regulation. Habilitationsschrift Mediz-
inische Fakultät der Charité Berlin
[37] Lasko T.A., Kripke D.F., Elliot J.A. (1999): Melatonin suppression by illumination of
upper and lower visual fields. J. Biol. Rhythms Vol. 14 Nr. 2 S. 122-125
[38] Lockley, S. W.; Brainard, G. C.; Czeisler, C. A. (2003): High Sensitivity of the Human
Circadian Melatonin Rhythm to Resetting by Short Wavelength Light. The Journal of Clinical Endocrinology & Metabolism 88(9): 4502-4505
[39] Lockley, S. W.: Light and Human Circadian Regulation, Proc. Symp. 2004 „Light &
Health" CIE x027: 2004, pp. 35-38, 2004
[40] Lucas, R. J.; Douglas, R. H.; Foster, R. G.: Characterization of an ocular photopig-
ment capable of driving pupillary constriction in mice, Nature Neurosci. 4, pp. 621-626, 2001
[41] Lucas, R. J.; Foster, R. G., Hankins, M. W.; Barnard, A. R.: Melanopsin, Photosensi-
tive Retinal Ganglion Cells and Non-Image Forming response to Light, Proc. Symp. 2004 „Light & Health" CIE x027: 2004, pp. 21-26, 2004
[42] Megdal, S.P., Kroenke, C.H., Laden, F., Pukkala, E., Schernhammer, E.S. (2005):
Night work and breast cancer risk: A systematic review and meta-analysis. European Journal of Cancer, Vol. 41 Nr. 13 S. 2023-2032.
[43] Melyan, Z.; Tarttelin, E. E.; Bellingham, J.; Lucas, R. J.; Hankins, M. W.: Addition of
human melanopsin renders mammalian cells photoresponsive, Nature 433, pp. 741-745, 2005
[44] Panda, S.; Nayak, S. K.; Cambo, B.; Walker, J. R.; Hogenesch, J. B.; Jegla, T.: Illumi-
nation of melatonin signaling pathway, Science 307, pp. 600-604, 2005
[45] Pauley, S.M. (2004): Lighting for the human circadian clock: recent research indicates
that lighting has become a public health issue. Medical Hypotheses, Vol. 63 Nr. 4 S.588-596.
[46] Qiu, X.; Kumbalasiri, T.; Carlson, S. M.; Wong, K. Y.; Krishna, V.: Provencio, I.; Ber-
son, D.: Induction of photosensitivity by heterologous expression of melatonin, Nature 433, pp. 745-749, 2005
[47] Rea, M. S.; Bullough, J. D.; Figueiro, M. G.; Bierman, A.: Spectral Opponency in Hu-
man Circadian Phototransduction: Implications for Lighting Practice, Proc. Symp. 2004 „Light & Health" CIE x027: 2004, pp. 111-115, 2004
[48] Rea, M. S.; Bullough, J. D.; Bierman, A,; Figueiro, M. G.: Implications for White Light
Sources of Different Correlated Color Temperatures. CIE Proc. Symp. „Lighting & Health" Ottawa CIE x032: 2006, pp. 33-38
[49] Revell, V. L; Burgess, H. L.; Gazda, C. J.;Smith, M. R.; Fogg, L. F.; Eastman, C. I.:
Advancing Human Circadian Rhythms with Afternoon Melatonin and Morning Intermit-tent Bright Light, J Clin Endocrinol Metab, January 2006, 91(1):54–59 55
[50] Rimmer, D.W., Boivin, D.B., Shanahan, T.L., Kronauer, R.E., Duffy, J.F., Czeisler,
C.A. (2000): Dynamic resetting of the human circadian pacemaker by intermittent bright light. American Journal of Physiology-Regulatory Integrative and Comparative Physiology, Vol. 279 Nr. 5 S. R1574-R1579
[51] Rüger, M. (2005): Lighting up the human circadian clock. Dissertation Universität
[52] Rüger, M.; Gordijn, M.C.M.; Beersma, D.G.M.; Vries, B.; Daan, S. (2005): Nasal ver-
sus temporal illumination of the human retina: effects on core body temperature, mela-tonin, and circadian phase. J of Biological Rhythms, Vol. 20 Nr. 1 S. 60-70
[53] Rüger, M., Gordijn, M.C.M., Beersma, D.G.M., de Vries, B., Daan, S. (2006): Time-of-
day-dependent effects of bright light exposure on human psychophysiology: compari-son of daytime and nighttime exposure. Am J Physiol Regul Integr Comp Physiol Vol. 290 S. R1413-20
[54] Sato, M.; Hiroki, N.; Takeshi, M.: The Effect of the Light with Different Spectral Ditribu-
tion During the Night Time on Melatonin Secretion and Psychological Factors in Hu-mans, Proc. Symp. 2004 „Light & Health" CIE x027: 2004, pp. 206-208, 2004
[55] Schernhammer, E.; Schulmeister, K.: Epidemiology of Night Work and Cancer Risk,
Proc. Symp. 2004 „Light & Health" CIE x027: 2004, pp. 46-49, 2004
[56] Smith, K. A.; Schoen, M. W.; Czeisler, C. A.: Adaptation of Human Pineal Melatonin
Suppression by Recent Photic History, J Clin Endocrinol Metab, July 2004, 89(7):3610–3614
[57] Stevens, R.G. (2006): Artificial lighting in the industrialized world: Circadian disruption
and breast cancer. Cancer Causes & Control, Vol. 17 Nr. 4 S. 501-507.
[58] Thapan, K.; Arendt, J.; Skene, D. J.: An action spectrum for melatonin suppression:
evidence for a novel non-rod, non-cone photoreceptor system in humans, J. Physiol. 535, pp. 261-267, 2001
[59] Wang J.Y., Hanifin J.P., Rollag M.D., Brainard G.C. (1999): Ocular regulation of the
human pineal gland: The significance of total retinal exposure for melatonin suppres-sion. Biologic Effects of Light 1998, Kluwer Boston, S. 367-373.
[60] Yoshimura, T.; Ebihara, S.: Spectral sensitivity of photoreceptors mediating phase-
shifts of circadian rhythms in retinally degenerate CBA/J (rd/rd) and normal CBA/N (+/+) mice, J. Comp. Physiol. [A] 178, pp. 797-802, 1996
[61] Zeitzer, J. M.; Dijk, D. J.; Kronauer, R. E.; Brown, E. N.; Czeisler, C.A.: Sensitivity of
the human circadian pacemaker to nocturnal light: melatonin phase resetting and sup-pression, Journal of Physiology 526.3, pp. 695-702, 2000
Source: http://www.m4ssl.npl.co.uk/wp-content/uploads/2012/02/Protocol-on-Circadian-effective-radiation.pdf
Pan Macmillan PPan Macmillan David Baldacci • The new heart-racing thriller from the Number One international best-selling author • David Baldacci is a consistent Sunday Times Top Five bestseller • He is the internationally-acclaimed author of 20 best-selling novels• His books are published in at least 45 different languages, and with over 90 million copies in print, he is one of the world's favourite storytellers.
Advances in Space Research 39 (2007) 1176–1181 Up-regulation of expression of tubulin genes and roles of microtubules in hypergravity-induced growth modification in Arabidopsis hypocotyls Shouhei Matsumoto, Yuka Saito, Saori Kumasaki, Kouichi Soga, Kazuyuki Wakabayashi, Takayuki Hoson * Department of Biology and Geosciences, Graduate School of Science, Osaka City University, Sumiyoshi-ku, Osaka 558-8585, Japan