Capa introdução _tese sabrina grassiolli_
SABRINA GRASSIOLLI
CONTRIBUIÇÃO DA FUNÇÃO DA CÉLULA BETA
NORMOGLICEMIA EM RATOS OBESOS – MSG.
Tese apresentada ao curso de Pós –
Graduação em Ciências Biológicas
(Área de Concentração – Biologia
Celular), da Universidade Estadual de
Maringá,
requisito
obtenção do grau de Doutora em
Ciências Biológicas.
CONTRIBUIÇÃO DA FUNÇÃO DA CÉLULA BETA
NORMOGLICEMIA EM RATOS OBESOS – MSG.
Orientador: Prof. Dr. Paulo Cezar de Freitas Mathias
Orientada: Sabrina Grassiolli
"Em toda luta por um ideal se tropeça por adversários e se criam
inimizades. O homem firme não os ouve e nem se detém a contá-los.
Segue sua rota irredutível em sua fé, imperturbável em sua ação,
porque quem marcha em direção de uma luz não pode ver o que ocorre
Autor desconhecido.
Tocando em Frente
Ando devagar porque já tive pressa
Levo esse sorriso porque já chorei demais
Hoje me sinto mais forte, mais feliz quem sabe
Só levo a certeza de que muito pouco eu sei, eu nada sei.
Conhecer as manhas e as manhãs
O sabor das massas e das maçãs
É preciso amor pra poder pulsar
É preciso paz pra poder sorrir
É preciso a chuva para florir.
Penso que cumprir a vida seja simplesmente
Compreender a marcha e ir tocando em frente
Como um velho boiadeiro
Levando a boiada eu vou tocando os dias
Pela longa estrada eu vou, estrada eu sou.
Conhecer as manhas e as manhãs
O sabor das massas e das maçãs
É preciso amor pra poder pulsar
É preciso paz pra poder sorrir
É preciso a chuva para florir.
Todo mundo ama um dia, todo mundo chora
Um dia a gente chega em outro vai embora
Cada um de nós compõe a sua história
Cada ser em si carrega o dom de ser capaz
de ser feliz.
Conhecer as manhas e as manhãs
O sabor das massas e das maçãs
É preciso amor pra poder pulsar
É preciso paz pra poder sorrir
É preciso a chuva para florir
Composição: Almir Sater e Renato Teixeira.
A
Deus no qual muitas vezes busquei as razões que me fizeram persistir em meio as
A
minha família, que é a razão de minha luta.
Ao meu noivo,
Marcelo pelo amor que sustentou e renovou as forças de minha jornada.
A cada um que conheci e principalmente aqueles com quem compartilhei conhecimentos e
emoções e que posso sem sombra de dúvidas dizer que são meus amigos:
Dionízia, pelos inarráveis momentos de alegria e pelas longas conversas…
Sandra, pelo exemplo de persistência, dignidade e cumplicidade…
Maroly, por ter fortalecido a minha fé…
Ana Eliza, pelo jeito honesto e sincero de manifestar suas idéias…
Fernanda Mesquita, pela irreverência e disponibilidade…
Rosana, pela persistência em buscar conhecimentos…
Anderson, pela fidelidade e apoio nos momentos difíceis…
Maria Lúcia, pela ajuda, atenção e apoio durante o desenvolvimento de meu trabalho…
Rodrigo, pela presença sempre alegre e descontraída…
Puzzi, através do qual ampliei meus conhecimentos sobre a alma feminina…
Diego, pela competência e dedicação…
Fernanda (maçã), pela coragem de mudar…
Aniela, pelo jeito meigo de lidar com as situações…
Fernando, pela inteligência e persistência…
Aos atuais colegas de laboratório:
Ricardo, Renato, Ângela, Marcelo, Tiara, Cíntia e
Juliana que partilharam dos momentos finais desse trabalho.
A
Clarice pelo apoio técnico.
Objetivo geral: Investigar os ajustes funcionais que asseguram a normoglicemia durante o desenvolvimento
da obesidade MSG.
Bases Teóricas: O equilíbrio do metabolismo corporal exige uma perfeita sintonia entre ações desencadeadas
pelo sistema nervoso central e endócrino. Uma das funções chaves para a homeostase é assegurar sob estrito
controle os níveis de glicose no plasma, o que é garantido pela liberação apropriada do hormônio insulina. Ao
longo do desenvolvimento surgem adaptações funcionais necessárias para garantir a manutenção desse
controle no intuito de adiar ou evitar a deteriorização do metabolismo. Desajustes na regulação da insulina e
conseqüentemente no perfil glicêmico são encontrados na obesidade. A crescente epidemia de obesidade é
acompanhada intimamente por uma inevitável elevação da prevalência de diabetes não dependente de insulina
(diabetes do tipo 2). A estreita ligação entre obesidade e diabetes, pode ser atribuído ao controle da secreção
de insulina pelas células beta. Durante a instalação da obesidade observa-se que a liberação de insulina pelas
células beta aumenta, proporcional a elevação da massa adiposa e da resistência periférica a insulina. Toda
vez que os ajustes metabólicos e funcionais realizados pelas células beta forem suficientes para sustentar a
demanda e superar a resistência periférica o controle dos níveis plasmáticos de glicose é garantido e mantido
em um novo ponto de ajuste. O tratamento neonatal com glutamato monossódico (MSG) induz em ratos
adultos obesidade, hiperinsulinemia e resistência periférica a insulina. Ilhotas pancreáticas de animais MSG
apresentam modificadas respostas à glicose e a neurotransmissores autonômicos. Apesar da obesidade
induzida por MSG reunir todos os elementos que predispõem ao surgimento do diabetes tipo 2, esta
anormalidade não aparece nestes animais.
Procedimento: Obesidade: Ratos Wistar machos receberam durante os 5 primeiros dias de vida injeções
subcutânea de MSG (4g/Kg de peso corporal). Controles (CON) receberam salina equimolar. Aos 21 dias os
animais foram desmamados e mantidos sob condições controladas de luminosidade (12h claro-escuro) e
temperatura (21± 2ºC). Estudos de desenvolvimento foram realizados em animais com 30, 60 e 90 dias. A
obesidade foi avaliada pelo cálculo do índice de Lee (IL) (peso corporal(g)1/3/comprimento naso-anal
(cm)x1000) e peso das gorduras periepididimal e retroperitoneal.
Teste de tolerância a glicose (ivGTT) e
insulina (ITT): Animais de ambos os grupos e idades receberam um cateter de silicone na veia jugular direita
e após 12 h de jejum foram submetidos a infusão de glicose (1g/Kg) ou insulina (1U/Kg). As amostras de
sangue retiradas durante o ivGTT e ITT eram utilizadas para dosagens da glicemia (oxidase-peroxidase) e
insulina (radioimunoensaio). O ivGTT também foi realizado na presença da acetilcolina (27nM/Kg). Análises
das áreas sob a curva (AUC) obtidas durante o ivGTT foram usadas para calcular o índice insulinogênico
(∆I/∆G), uma medida da resistência periférica a insulina. Após a injeção de insulina a razão de
desaparecimento da glicose (Kitt/min) foi avaliada.
Ilhotas pancreáticas: Ilhotas pancreáticas foram isoladas
pelo método da colagenase e incubadas por 60min com glicose (2,8-28,8mM) ou glicose 8,3mM na presença
de diferentes concentrações de acetilcolina (0,001 - 3000µ M). As vias dependentes e independentes da glicose
sobre canais de K+ATP foram estudadas em meio com glicose 16,7mM e/ou diazoxida (250µM) e solução de
potássio elevada (30mM). A participação da acetilcholina via receptor muscarínico subtipo M2 foi testada em
meio contendo glicose 8,3mM na presença de acetilcolina (10µM) mais antagonista muscarinico M2
metoctramine – MTT - (1, 5 e 10µM).
Transplante de Ilhotas: 600 ilhotas isoladas de ratos magros e obesos
foram transplantadas via veia porta-hepática em ratos diabéticos. A glicemia foi monitorada durante 4 dias
após o transplante. Depois de 12 h de jejum os animais transplantados foram sacrificados, amostras de sangue
foram colhidas para dosagem da glicemia e insulinemia. A gordura retroperitoneal foi retirada e pesada.
Analise Estatística: Dados foram expressos como media ± erro padrão da média. Teste t de Student's foi
usado para comparação entre dois grupos e análise de variância (ANOVA) para mais de 2 grupos. *p<0,05 foi
adotado como critério de significância.
Resultados: O IL foi em média 9% maior em animais MSG comparados aos CON nas mesmas idades. O
desenvolvimento afetou de forma similar o IL em ambos os grupos, sendo aproximadamente 5% menor em
animais aos 60 dias, comparados aos 30 e 90 dias. As gorduras periepididimal e retroperitoneal foram em
média 100% maiores em MSG aos 30, 60 e 90 dias em relação aos CON nas mesmas idades. O
desenvolvimento elevou o conteúdo de gordura em ambos os grupos, todavia a magnitude do efeito foi maior
em animais MSG. Enquanto a glicemia de jejum praticamente não foi afetada pelo tratamento com MSG ou
pelo desenvolvimento, os níveis plasmáticos de insulina variaram para os dois fatores. Animais MSG aos 60 e
90 dias apresentam cerca de 6 e 3 vezes mais insulina, quando comparados aos respectivos CON. O
desenvolvimento elevou em 4 vezes a insulina de jejum de ratos CON dos 30 aos 90 dias; em MSG este efeito
foi progressivo aumentando 6 vezes e 12 vezes respectivamente aos 60 e 90 dias comparados aos 30 dias.
Embora a AUC da glicose e da insulina obtidas durante o ivGTT tenham sido maiores em MSG 47 e 160%
respectivamente em relação aos CON nas 3 idades avaliadas; o perfil desses parâmetros foi o mesmo em
ambos os grupos durante o desenvolvimento. A AUC da glicose não mudou enquanto a AUC da insulina
apresentou um aumento de 4 vezes em ratos CON dos 30 dias aos 90 dias e 10 vezes em MSG no mesmo
período. A resistência à insulina foi 78 e 112% maiores em ratos MSG aos 60 e 90 dias em relação aos CON
nas mesmas idades, confirmado também pela menor Kitt 36% em animais MSG adultos. Ilhotas pancreáticas
apresentaram típica curva dose resposta a glicose e a acetilcolina nas 3 idades avaliadas para ambos os grupos.
Todavia, ilhotas de ratos MSG liberam aproximadamente 2 vezes mais insulina em resposta e elevadas
concentrações de glicose (8,3 – 16,7mM); embora não apresentem diferenças na resposta a baixas
concentrações de glicose (2,8-5,6mM). Apenas aos 60 dias a sensibilidade a glicose de ilhotas pancreáticas de
MSG foi 12% maior em relação aos CON na mesma idade. A resposta secretora de ilhotas pancreáticas a
glicose foi maior para baixas concentrações em animais jovens e para altas concentrações em animais adultos.
Apesar dessas diferenças a sensibilidade à glicose foi similar para todas a idades em animais CON. Em ratos
MSG a sensibilidade foi 13% maior em animais aos 60 dias comparados aos 30 e 90 dias. Ilhotas de animais
CON e MSG apresentam redução de 85% na resposta a glicose em presença de diazoxida, todavia os efeitos
de elevadas concentrações de K+/diazoxida existiram apenas em ilhotas de ratos CON. Embora o
desenvolvimento não afete a sensibilidade a acetilcolina em ilhotas de ambos os grupos, em ratos MSG existe
uma queda de aproximadamente 85% na resposta máxima a acetilcolina nas 3 idades avaliadas comparados
aos respectivos controles. Ilhotas de ratos adultos apresentaram 50% de redução na resposta máxima a
acetilcolina comparados aos jovens. Apenas em ilhotas de MSG o antagonista MTT potencializou o efeito da
acetilcolina em cerca de 120%. O transplante de ilhotas pancreáticas foi eficiente em normalizar a glicemia de
jejum de animais diabéticos independente da origem dessas ilhotas.
Conclusões:
► Durante o desenvolvimento elevam-se simultaneamente o conteúdo de gordura corporal, os níveis
circulantes de insulina e a resistência periférica, todos os parâmetros são profundamente acentuados pelo
tratamento neonatal com MSG. ► As respostas secretoras de ilhotas pancreáticas se ajustam ao estado fisiológico sendo mais responsivas em ratos MSG nos quais a resistência à insulina e o acúmulo de gordura são mais elevados. ► O desenvolvimento não afeta a sensibilidade a glicose ou ao seu principal potenciador acetilcolina, todavia altera marcantemente as respostas secretoras máximas aos dois efeitos. ► Ilhotas pancreáticas de animais MSG passam ao longo do desenvolvimento por mudanças no controle secretor, ocorre inicialmente aumento da sensibilidade a glicose e posteriormente aumento da capacidade
secretora e conseqüentemente normalização da sensibilidade a glicose, comparado aos animais magros. ► A ação da glicose em canais de K+ATP está intacta em ilhotas de MSG-obesos, todavia os efeitos amplificadores do secretagogo estão ausentes. ► Os efeitos da acetilcolina sobre a secreção de insulina estimulada por glicose em ilhotas pancreáticas também sofrem modulações ao longo do desenvolvimento, preserva-se a sensibilidade em detrimento da
resposta máxima ao agonista. ► Ilhotas pancreáticas de MSG-obesos apresentam similaridades para a resposta a acetilcolina de ilhotas de animais magros durante o desenvolvimento , todavia em todas as idades a magnitude do efeito da acetilcolina
está reduzida. ► A diminuição do efeito da acetilcolina em ilhotas de animais MSG poder ser atribuída a uma reorganização e/ou função dos receptores muscarínicos, esta hipótese é reforçada pela observação de um efeito inibitório da
acetilcolina mediado por receptores muscarínicos do subtipo M2. ► As adaptações funcionais das ilhotas pancreáticas dos animais obesos não são permanentes, e podem ser revertidas como mostrado pelo transplante.
ABSTRACT
GENERAL AIM: To investigate functional readjustments that guarantee normoglycemia
during the development of MSG obesity.
Theoretical bases: Equilibrium in body metabolism requires a perfect harmony among the
activities triggered by central and endocrine nervous system. Homeostasis's key functions
strictly ensure glucose levels in the plasma which is warranted by the adequate release of
insulin. Necessary functional adaptations occur throughout development and sustain control
so that the metabolism's deterioration could be postponed or avoided. Non-adjustments to
insulin control, or rather, a glycemic condition, are proper to obesity. Current obesity
epidemic is followed by an inevitable rise in non-dependent insulin diabetes or Type 2
diabetes. Close coupling between obesity and diabetes is a result of the control of insulin
secretion by beta cells. There is an increase in insulin release by beta cells during the onset
of obesity proportional to adiposity mass and to peripheral resistance to insulin. When
metabolic and functional adjustments by beta cells are sufficient to sustain demand and
peripheral resistance is overcome, control of glucose plasma levels is guaranteed and kept
at a new adjustment level. Monosodium glutamate (MSG) neonatal treatment induces
obesity, hyperinsulinemia and peripheral resistance to insulin in rats. MSG animals'
pancreatic islets show modified responses to glucose and to autonomous neurotransmitters.
Although MSG-caused obesity has all the factors that predispose the subject to Type 2
diabetes, the abnormality does not occur in these animals.
Procedure.
Obesity: Male Wistar rats were subcutaneously injected with MSG (4g/kg body
weight) during the first five days of life. Controls (CON) received an equimolar saline
solution. Animals were weaned after 21 days and maintained under light control (12h light-
dark) and temperature (21±2°C) conditions. Development studies were undertaken with 30-,
60- and 90-day-old animals. Obesity was assessed by Lee Index (LI), body weight
(g) /nasal-anal length (cm)x1000) and weight of periepidydimal and retroperitoneal fats.
Tolerance glucose (inGTT) and insulin (ITT) test: A silicon catheter was introduced in the
right jugular vein of animals of the two groups and age; after 12h fasting they underwent
glucose (1g/kg) or insulin (1U/kg) infusion. Blood samples, removed during ivGTT and
ITT, were deployed for glycemia (oxydase-peroxydase) and insulin (radioimmunoassay)
dosages. ivGTT was also undertaken with acetylcholine (27nM/kg). Analyses of areas
under curve (AUC), obtained during ivGTT, were used to calculate insulinogenic index
(∆1/∆G) and measure peripheral resistance to insulin. Ratio of glucose disappearance
(Kitt/min) after insulin injection was evaluated.
Pancreatic islets: pancreatic islets were
isolated by collagen method and incubated for 60min with glucose (2.8-28.8mM) or
glucose 8.3mM with different acetylcholine concentrations (0.001 – 3000µM). Glucose
dependent and independent pathways on K+ATP were studied in a medium composed of
16.7mM glucose and/or diazoxide (250µM) and high potassium solution (30mM). The
participation of acetylcholine through subtype M2 muscarinic receptor was tested in a
medium composed of 8.3mM glucose with acetylcholine (10µM) plus antagonistic M2
muscarinic metoctramine – MTT – (1, 5 and 10µM).
Transplantation of islets: 600 isolated
islets of lean and fat rats were transplanted in diabetic rats through the hepatic vein.
Glycemia was monitored during 4 days after transplantation. After 12h fasting the
transplanted animals were killed and blood samples were taken for glycemia and
insulinemia dosages. Retroperitoneal fat was removed and weighed.
Statistical analysis:
Data were given as mean ± mean standard error. Student's t test was used to compare the
two groups, while variance analysis (ANOVA) compared more than two groups; *p<0.05
was significant.
Results. On average LI was 9% higher in MSG animals when compared to CONs of the
same age bracket. Development influenced LI of the two groups similarly, approximately
5% less in 60-day-old animals when compared to 30- and 90-day-old ones. Periepididimal
and retroperitoneal fats were, on an average 100% higher in 30-, 60-, and 90-day-old MSG
rats when compared to CONs of the same age bracket. Although development raised fat
contents in both groups, it was higher in MSG animals. While fasting glycemia was
practically unaffected by MSG treatment or by development, plasma levels of insulin
varied for the two factors. 60- and 90-day-old animals had approximately 6 and 3 times
more insulin when compared to respective CONs. Development raised 4 fold fasting insulin
of 30-day-old to 90-day-old CON rats. In MSG ones the effect increased progressively 6
and 12 fold respectively in 60- and 90-day-old rats when compared to 30-day-old ones.
Although glucose and insulin AUC obtained during ivGTT were higher in MSG rats by 47
and 160% respectively when compared to those of CONs in the three age brackets under
analysis, parameters were the same in the two groups during development. Whereas
glucose AUC did not change, insulin AUC increased 4 fold in CONs within 30 to 90 days
period and 10 fold in MSGs during the same period. Insulin resistance was 78 and 112%
higher in 60- and 90-day-old MSG rats when compared to CONs of the same age. This is
corroborated by the least Kitt, 36% in adult MSG rats. Pancreatic islets had a typical dose
curve to glucose and to acetylcholine in the three ages under analysis for the two groups.
However, islets of MSG rats released approximately 2 fold more insulin and high
concentrations of glucose (8.3 – 16.7mM), even though there were no differences in
response to low glucose concentrations (2.8 – 5.6mM). After 60 days sensitivity to glucose
of MSG rats' pancreatic islets was 12% higher when compared to that in CONs of the same
age. Secretory response of pancreatic islets to glucose was higher for low concentrations in
young animals and for high concentrations in adult animals. In spite of the above difference,
glucose sensitivity was similar in CONs at all ages. Sensitiveness in MSG animals was
13% less in 60-day-old animals when compared to that in 30- and 90-day-old ones. Islets of
CON and MSG animals had an 85% reduction in response to glucose when diazoxide was
present. However, effects of high concentrations of K+/diazoxide were present only in CON
rats. Although development does not affect sensitiveness to acetylcholine in islets of both
groups, an approximately 85% decrease has been reported in MSG rats as maximum
response to acetylcholine at the three ages evaluated when compared to their respective
CONs. Adult rats' islets had a 50% decrease in their maximum response to acetylcholine
when compared to that in young ones. Only in MSG rats' islets did antagonist MTT
potentialize acetylcholine effect to about 120%. Transplantation of pancreatic islets was
sufficient to normalize fasting glycemia in diabetic animals regardless of the islets' origin.
Endocrine, vol. 30, no. 2, 000–000, October 2006 0969–711X/06/30:000–000/$30.00
ENDO (Online) ISSN 1559-0100 2006 by Humana Press Inc.
All rights of any nature whatsoever reserved.
Pancreatic Islets from Hypothalamic Obese Rats
Maintain K+ Channel-Dependent but Not -Independent
Pathways on Glucose-Induced Insulin Release Process
Sabrina Grassiolli, Maria Lúcia Bonfleur, Dionizia Xavier Scomparin,and Paulo Cezar de Freitas Mathias
Laboratory of Secretion Cell Biology, Department of Cell Biology and Genetics, State University of Maringá,Maringá, PR, Brazil
One of the main features of obesity is hyperinsulinemia,
which is related to insulin oversecretion. Glucose is by
Glucose is by far the most important physiological regu-
far the major physiological stimulator of insulin secre-
lator of pancreatic beta cells' functions. The ATP/ADP ratio
tion. Glucose promotes an increase in the ATP/ADP
is understood to couple glucose metabolism with the closing
ratio, which inactivates ATP-sensitive K+ channels (K+
of ATP-sensitive K+ (K+ATP) channels and the ionic events "(K+
and induces beta cell depolarization with consequent
leading to the exocytosis of insulin (1). The mechanisms by addition
calcium influx. Increased intracellular calcium concen-
which glucose controls pancreatic beta cells' function are
tration triggers insulin exocytosis. K+
ATP channel func-
complex (2,3). A number of studies have established that
tion is important for K+ATP channel-dependent path-
a metabolic control of ionic events in beta cells is a critical
ways involved in glucose-stimulated insulin secretion
step in secretion–stimulus coupling (4), and assigned the
(GSIS). However, K+ATP channel-independent pathway
K+ATP channel in the plasma membrane a pivotal role in this
has been identified and it has been found that this
control (5). The essential role of K+ATP channels is strikingly
pathway sustains GSIS. Both pathways are critical to
illustrated by the inhibition of insulin release brought about
better GSIS control. GSIS was studied in pancreatic
by diazoxide. This drug selectively opens K+ATP channels
islets from hyperinsulinemic adult obese rats obtained
and does not cause depolarization of the plasma membrane
by monosodium L-glutamate (MSG) neonatal treatment.
or inhibition of Ca2+ influxes; the metabolism of glucose is
Islets from MSG-obese rats were more glucose respon-
unchanged (6). There is evidence that glucose can control
sive than control ones. Diazoxide, a drug which main-
insulin release independently from its action on K+ATP chan-
tains the K+ATP channels open without interfering with
nels (7). A second pathway of regulation by glucose is tested
cell metabolism, blocked GSIS in islets from both groups.
by treating beta cells with diazoxide to hold K+ATP channels
High extracellular potassium concentration plus diaz-
open along with a high extracellular K+ concentration that
oxide was used to study an alternative to the K+ATP chan-
depolarizes the membrane and restores Ca+2 influx. This
nel pathway; in these conditions islets from MSG-obese
condition does not allow glucose to induce increased intra-
rats did not respond, while islets from control animals
cellular calcium ([Ca+2]i), but amplifies the action of the
showed enhanced GSIS. Results indicate that MSG-obese
ion on the insulin-releasing process (8).
rats oversecreted insulin, even though the K+ATP chan-
Obesity is associated with abnormal control of insulin se-
nel-independent pathway is impaired in their beta cells.
cretion in animals and humans. Hyperinsulinemia and periph-eral insulin resistance are characteristic of human and ani-
Key Words: Pancreatic islets; MSG obese rats; K+ATP
mal obesity. Although the precise mechanisms that cause
channels; insulin release; diazoxide.
obesity are not yet understood, beta cells' functions are im-paired and glucose-induced insulin release is enhanced inanimal experimental obesity models (9,10). Studies in obesehyperinsulinemic rodents, ob/ob mice, and Zucker rats, orin GK rats, who present non-insulin-dependent diabetes mel-litus (NIDDM) showed that their beta cells do not display
Received July 17, 2006; Revised September 2, 2006; Accepted October 2,
any alteration in K+ATP channel function (11,12). These
studies suggest that insulin oversecretion cannot be attrib-
Author to whom all correspondence and reprint requests should be addressed:
uted to K+ATP channel dysfunction of beta cells from obese
Paulo Cezar de Freitas Mathias, State University of Maringá, Department
animals. In the presence of diazoxide and a depolarizing
of Cell Biology and Genetics, Avenida Colombo 5790, Maringá, PR, Brazil87020-900. E-mail:
[email protected]
extracellular concentration of potassium, glucose was still
Insulin Release and K+ Channels in MSG Rats / Grassioli et al.
Effects of Neonatal Treatment with MSG
Basal insulin (ng/mL)
Basal glycemia (mM)
Periepididymal fat (g/100g bw)
Retroperitoneal fat (g/100g bw)
Data present media ± SEM. Eight to 10 animals from both groups were used
to obtain these results. The glucose and insulin blood levels were evaluated afterfasting overnight. Data from fat pads were expressed in relation to body weight.
Differences were analyzed by Student's t test (*p < 0.001).
Fig. 1. Glucose tolerance test (ivGTT). Each symbol represents the mean from 8 to 10 animals from both groups. Lines over the symbolrepresent the SEM. The increment of glycemia is shown in panel A and insulinemia in panel B; both data were obtained after glucoseload (1 g/kg). Differences were analyzed by Student's t test (*p < 0.05).
able to induce insulin secretion in pancreatic islets from lean
pared to untreated rats (p < 0.001). Fasting blood levels of
rats and humans (13,14). Even in islets from obese ob/ob
insulin were 400% highest in MSG rats than in control
mice and Zucker rats, the alternative pathway remained
animals (p < 0.001). In spite of higher levels of insulin,
intact (15,16). However, in islets from diabetic GK rats,
MSG rats presented blood glucose levels similar to control
reduced activity of this K+ATP channel-independent path-
way was observed (17,18).
Glucose Tolerance Test
Monosodium L-glutamate (MSG) administered during the
neonatal period induces obesity with marked hyperinsulin-
The increment of glycemia is presented in Fig. 1A, while
emia and insulin resistance in adult animals (19). These
the insulin increment is shown in Fig. 1B. The ivGTT is a
major abnormalities have been attributed to a disruption in
technique that simulates the fed state and reveals that MSG
the insulin-secretion control in pancreatic islets. Islets iso-
rats are glucose intolerant. Plasma glucose increments were
lated from MSG rats presented higher insulin release when
154% higher in MSG rats when compared to control animals
incubated in the presence of elevated glucose concentra-
(p < 0.001), throughout the ivGTT. Blood insulin levels
tion. In spite of presenting higher fasting levels of insulin
were 70% higher at 2, 5, 10, and 15 min, compared to the
in plasma, MSG rats are normoglycemic (20). Furthermore,
same times in control animals (p < 0.001).
these obese adult animals are not considered diabetic. The
Insulin Secretion in Isolated Pancreatic Islets
purpose of the present work is to evaluate the K+ATP channel-dependent and -independent pathways on glucose-induced
insulin secretion in pancreatic islets isolated from MSG-
Glucose exerted more evident insulinotropic effect in
islets from MSG rats. The release of insulin was 40% greater,with 8.0–16.7 mM of glucose in islets from MSG rats, than
in the control ones ( p < 0.001), as shown in Fig. 2.
Effects of Neonatal Treatment with MSG in Adult Rats
Diazoxide Response
Table 1 shows that MSG rats presented a 152% increase
Diazoxide 250 µM, a K+ATP channel opener, which avoided
in the periepididymal and retroperitoneal fat pad when com-
depolarization of beta cells, inhibited by 85% the glucose
Vol. 30, No. 2
Insulin Release and K+ Channels in MSG Rats / Grassioli et al.
Fig. 2. Insulin secretion by islets in different glucose concentrations. Each symbol presents the mean from 20 batches of four isletsincubated for 60 min in 1 mL of medium containing different glucose concentrations. Lines over the symbol represent the SEM.
Differences were analyzed by Student's t test (*p < 0.05).
Fig. 3. Effects of diazoxide and high K+ on induced insulin secretion in isolated pancreatic islets. Batches of four islets were incubatedfor 60 min in 1 mL of medium containing glucose, K+, and diazoxide, explained in Methods. Values are mean ± SEM for 20 batches ofislets. Panel A presents the effects in islets from lean rats and panel B shows the effects in islets from MSG-obese rats. The letters overthe bars represent significant (ANOVA) differences with p < 0.05 between: a, glucose; b, glucose + diazoxide; c, glucose + K+, and d,glucose + K+ + diazoxide.
16.7 mM response in pancreatic islets from both animal groups
cose 16.7 mM alone in islets from control rats (p < 0.001).
(Figs. 3A,B). The effect of diazoxide completely eliminated
The stimulatory effect of high potassium upon insulin secre-
glucose response when compared to the secretion induced
tion was not observed in islets from MSG rats, as shown in
by glucose 5.6 mM, as shown in Fig. 2.
High Extracellular Potassium Response
Glucose Response in the Presence of Diazoxide
High extracellular potassium concentration (30 mM)
and High Extracellular Potassium
impaired K+ out fluxes and induced depolarization in beta
With the K+ATP channel opened by diazoxide and inacti-
cells. Under these conditions (Fig. 3A), insulin secretion
vation of other K+ channels by 30 mM of KCl, glucose 16.7
was increased by 55% when compared to the effect of glu-
mM was able to increase insulin secretion by 120% and 43%
Insulin Release and K+ Channels in MSG Rats / Grassioli et al.
when compared to glucose alone and glucose in the presence
manner similar to that shown by islets from lean normal
of high potassium, respectively (p < 0.01), in islets from
rodents (16,39). Glucose-induced insulin secretion was
control rats. The same experimental conditions did not
inhibited by diazoxide 250 µM in islets of MSG-obese rats
affect the insulin secretion stimulated by glucose or glu-
with the same magnitude as in the islets of lean rats (16). It
cose plus high potassium in islets from MSG-rats, as shown
has been established that 250 µM of diazoxide concentra-
tion is enough to ensure that all K+ATP channel are opened,preventing the insulin exocytosis stimulated by glucose.
Our results indicate that the insulin secretion is also greatly
dependent on K+ATP channel function in islets of MSG-
Neonatal treatment with MSG caused obesity in adult
obese rats. As in other obese rodents, the insulin oversecre-
rats with 90 d of age, as estimated by fat accumulation in
tion could be related to abnormalities in glycolytic pathways
epididymal and retroperitoneal pads. Hyperinsulinemia
more than in K+ATP channel dysfunctions.
was also observed in MSG-obese rats, without high blood
High K+ membrane permeability is very important to
glucose concentration. MSG treatment lesioned a major part
determine the resting potential of beta cells. Although this
of localized in the hypothalamic arcuate nucleus (ARC),
permeability decreases when glucose concentration is raised,
disarranging an important area involved in body weight
the membrane potential of beta cells remains very sensitive
control (21). Obese hyperinsulinemic rodents having a
to changes in the equilibrium potential of K+. Increasing
genetic defect, such as Zucker rats and ob/ob mice, present
extracellular potassium moves the equilibrium potential of
with hyperphagia (22), while MSG-obese rats show normo-
K+ to less-negative values, which leads a sustained depolar-
or hypophagia (19,23). However, MSG-rats accumulate
ization. Under these conditions, [Ca+2]i and insulin release
increased fat in tissues (24,25). Up to 210 d of age, MSG-
are increased, as observed in the blockage of K+ATP channel
obese animals do not develop hyperglycemia, while pre-
(40). As expected, high extracellular potassium enhanced
senting insulin resistance (26,27). MSG-obese rats present
the glucose-induced insulin release in islets from lean rats;
with fasting and fed hyperinsulinemia, as evoked during
however, the effect of depolarization with high potassium
the ivGTT test. The same results were obtained by other
was not recorded in islets from MSG-obese rats. Our results
authors (28,29).
suggest that beta cells from MSG-obese rats have abnor-
Hyperinsulinemia in MSG-obese rats has been attributed
malities in the oscillations of membrane potential, when this
to insulin oversecretion (20,30). As we showed, pancreatic
islets are stimulated by glucose, as showed also in ob/ob
islets from MSG-obese rats are more responsive to high
glucose concentrations than islets from lean rats. However,
It has been demonstrated that insulin release stimulated
at glucose 5.6 mM, which has been considered the glucose
by glucose can bypass the K+ATP channel pathway. Islets
concentration of fasting, the stimulated insulin release was
from normal mice, rats, and humans treated with diazoxide
similar in both islet groups. Other factors, such as high para-
in the presence of depolarizing extracellular potassium con-
sympathetic activity, have been suggested to be involved
centrations still secrete insulin when stimulated by glucose.
in the cause of hyperinsulinemia in fasting, observed in
In these conditions, the magnitude of the secretion is high
MSG-obese rats (20). Islets isolated from Zucker rats and
in comparison with glucose insulinotropic effect by itself
ob/ob mice showed increased insulin release when stimu-
or glucose combined with high extracellular potassium con-
lated by low and high glucose concentrations (31,32). A
centration (13,14,42). These results support the suggestion
higher response to glucose has also been observed in islets
that glucose-induced insulin secretion is a resultant from
isolated from hypothalamic obese rats, such as those lesioned
pathway-dependent and -independent K+ATP channel func-
in the ventromedial area (VMH) (33). Much experimental
tions. However, the mechanisms by which glucose can con-
evidence supports the proposal that glucose stimulates the
trol insulin release independently from changes in K+ATP
beta cell metabolism to trigger mechanisms that lead to the
channel activity and changes in beta cells' membrane poten-
exocytosis of insulin granules (34–37). It can be suggested
tial requires stimulation of beta cells' metabolism, but does
that, independently of their origin, experimental obese
not involve increases in intracellular calcium, cAMP lev-
rodents present alterations in the regulation of glucose-in-
els, inositol phosphate levels, or PKC activity (7). Alterna-
duced insulin secretion, at least partly as a result of changes
tively, ATP/ADP ratio controls insulin release by regulat-
in early stages of the glycolytic pathway. ATP levels have
ing both the activity of K+ATP channel and a much later step
been known to couple glucose metabolism with the closing
of stimulus-secretion coupling. The latter pathway seems
of K+ATP channel. The resulting decrease in potassium con-
to be related to the effect of glucose on the amplification of
ductance leads to depolarization of the membrane with sub-
the effectiveness in intracellular calcium rise, which was
sequent opening of voltage-dependent calcium channels.
provoked by the K+ATP channel pathway. The possibility that
An increase in intracellular Ca2+ starts the insulin exocyto-
insulin oversecretion, observed in obesity, could be related
sis (38). The islets from obese Zucker rats and ob/ob mice
to K+ATP channel alternative pathway in glucose-induced
respond to diazoxide and blockade of K+ATP channels in a
insulin secretion was tested in islets of MSG-obese rats.
Vol. 30, No. 2
Insulin Release and K+ Channels in MSG Rats / Grassioli et al.
Our results confirmed that islets isolated from lean rats
obtained was filtered with a 0.5 mm metal mesh and washed
used this pathway, while islets of MSG-obese did not. Islets
with HBSS, including 0.12% bovine serum albumin frac-
from obese ob/ob mice and Zucker rats have no changes
tion V (BSA) in five continuous washings. The islets were
in this mechanism (15,16). However, islets from diabetic
collected with the aid of a microscope. At least three rats
GK rats, which present a reduction in glucose-induced insu-
were used to obtain a pancreatic rat pool for each animal
lin release, showed a decrease in K+ATP channel alternative
pathway function (11). Even though MSG-obese rats pres-
Islets Incubation
ent hyperinsulinemia and normoglycemia, their pancreaticislets showed normal functioning of the K+
Batches of four islets were preincubated in the presence
ATP channel path-
way, but absence of function in K+
of 1 mL of normal Krebs solution containing 120 mM NaCl;
ATP channel-independent
pathway. Alternatively, to solve this paradox, it is possible
4.8 mM KCl; 2.5 mM CaCl2; 1.2 mM MgCl2, and 24 mM
to speculate that beta cells from MSG-obese rats have en-
NaHCO3. This solution was gassed with O2/CO2 (95/5%)
hanced glycolytic metabolism, allowing a decrease of the
to maintain pH 7.4 and supplemented with bovine albumin
threshold for glucose-stimulated insulin release, through the
fraction V (0.12%) for 60 min in the presence of glucose
5.6 mM. After this period, the islets were submitted for
ATP channel pathway function. Patients with hyperinsu-
linemia caused by mutations in glycolytic enzymes respond
another 60 min of incubation in glucose stimulatory con-
well to diazoxide (43,44).
dition (16.7 mM) with normal or high K+ (30 mM) Krebs
Regardless of the evidence that for precise control of
solution. Both experimental conditions were also evaluated
insulin blood levels, beta cell must to set triggering path-
in the presence or absence of diazoxide (250 µM). When
to set." ways (dependent K+
concentration of KCl was increased to 30 mM, NaCl concen-
ATP channel) and amplifying pathways
tration was decreased to 94.8 mM to maintain isoosmolar-
ATP channel) in glucose-stimulated insulin
release process, our findings indicate that pancreatic islets
ity. In another protocol, the islets were submitted to 60 min
isolated from MSG-obese rats are able to sustain insulin
of incubation in the presence of normal Krebs with differ-
oversecretion, even without to use K+
ent glucose concentrations (5.6, 8.0, 11.1, 16.7, and 20.0
ATP channel-indepen-
dent pathway.
mM). Samples of incubation media were taken and storedfrozen until assay to measure the secreted insulin by radio-immunoassay (RIA) (46).
Materials and Methods
Glucose Tolerance Test
Animals and Obesity
Under anesthesia, a silicone cannula was implanted into
Neonate Wistar rats received monosodium-L-glutamate
the remaining rats' left jugular and attached to the animals'
(MSG) injections intradermally during the 5 first days of
back. To avoid blood clotting, heparinized saline (50 IU
life, with a dose of 4 g/kg of body weight (BW). Control
heparin in saline 0.9%/mL) was previously injected into
animals received equimolar saline solution. Both groups
the cannula. Intravenous glucose tolerance tests (ivGTT)
of animals were weaned at 21 d of age. All animals were
were performed at 8:00 after a 12 h fast (19:00–07:00) and
housed under control conditions of the luminosity cycle of
without any anesthesia rats were injected with a glucose
12 h light–dark (07:00 to 19:00 h) and temperature of 21 ±
load (1 g glucose/kg BW) through the cannula. Blood sam-
2°C. Water and standard rodent chow (Nuvital-Curitiba-
ples (300 µL) were collected from the same cannula sequen-
Brazil) were supplied ad libitum. Only males were used in
tially before glucose load (t 0) and 5 (t5), 10 (t10), 15 (t15),
the experimental protocols. At 90 d, rats from both groups
20 (t20), 25 (t25), and 30 (t30) min after the injection of
were anesthetized with ketamine and xylasine (55 and 8
glucose (47). They were then centrifuged, and the plasma
mg/kg BW, respectively) and sacrificed by cervical dislo-
was separated and stored at 20°C for posterior dosage of glu-
cation. Periepididymal and retroperitoneal fat pad were
cose concentration by the glucose oxidase method (Kit-Bio
removed, washed, and weighed to estimate the obesity
Diagnostic Chemistry Industry®) and insulin by RIA. The
induced by MSG treatment.
State University of Maringá Ethical Committee for Animal
Pancreatic Islets Isolation
Experiments approved the described protocols.
Isolation of islets from the rats' pancreas was performed
as previously described (45) with adaptations. Rats from
[125I]Insulin human recombinant was acquired from Phar-
another sample were anesthetized had their abdominal wall
macia (São Paulo, BR). Routine reagents were purchased
cut and open. A 10 mL Hank's buffered saline solution
from Sigma unless otherwise specified.
(HBSS) containing collagenase type V (0.7 mg/mL, SigmaChemical Co., St. Louis, MO) was injected into the com-
Statistical Analysis
mon bile duct of the rats. The pancreas, swollen with the
Results were given as mean ± SEM. Data were submit-
collagenase solution, was quickly excised and incubated in
ted to Student's t test or variance analysis (ANOVA). In
a plastic culture bottle for 15 min at 37°C. The suspension
the case of analyses with a significant F, the differences
Insulin Release and K+ Channels in MSG Rats / Grassioli et al.
between means were evaluated by Bonferroni t test. p values
22. Bray, G. A. (1991). J. Nutr. 121, 1146–1162.
less than 0.05 were considered statistically significant. The
23. Dawson, R. Jr., Wallace, D. R., and Gabriel, S. M. (1989).
Pharmacol. Biochem. Behav. 32, 391–398.
tests were performed using GraphPad Prism version 3.02
24. Nascimento Curi, C. M., Marmo, M. R., Egami, M., Ribeiro,
for Windows (GraphPad Software®).
E. B., Andrade, I. S., and Dolnikoff, M. S. (1991). Biochem.
Int. 24, 927–935.
25. Macho, L., Fickova, M., and Jezovaand Zorad, S. (2000).
Physiol. Res. 49 (Suppl. 1), S79–85.
26. Kim, Y. W., Kim, J. Y., and Lee, S. K. (1999). J. Korean Med.
We gratefully acknowledge financial support CNPq (Bra-
Sci. 14, 539–545.
zilian Council for Scientific and Technological Develop-
27. Hirata, A. E., Alvarez-Rojas, F., Carvalheira, J. B., et al. (2003).
Life Sci. 73, 1369–1381.
28. Hirata, A. E., Andrade, I. S., Vaskevicius, P., and Dolnikoff,
M. S. (1997). Braz. J. Med. Biol. Res. 30, 671–674.
29. de Mello, M. A., de Souza, C. T., Braga, L. R., dos Santos,
J. W., Ribeiro, I. A., and Gobatto, C. A. (2001). Physiol. Chem.
1. Sweet, I. R., Cook, D. L., DeJulio, E., et al. (2004). Diabetes
Phys. Med. NMR 33, 63–71.
53, 401–409.
30. Marçal, A. C., Grassiolli, S., Rocha, D. N., et al. (2006). Endo-
2. Henquin, J. C., Ravier, M. A., Nenquin, M., Jonas, J. C., and
crine 29, 445–449.
Gilon, P. (2003). Eur. J. Clin. Invest. 33, 742–750.
31. Chan, C. B., Lowe, J. M., and Debertin, W. J. (1996). Int. J.
3. Ashcroft, F. M. (2005). J. Clin. Invest. 115, 2047–2058.
Obes. Relat. Metab. Disord. 20, 175–184.
4. Malaisse, W. J. (1992). Int. J. Biochem. 24, 693–701.
32. Chen, N. G., Tassava, T. M., and Romsos, D. R. (1993). J. Nutr.
5. Ashcroft, F. M., Harrison, D. E., and Ashcroft, S. J. (1984).
123, 1567–1574.
Nature 312, 446–448.
33. Campfield, L. A., Smith, F. J., and Eskinazi, R. E. (1984). Am.
6. Dunne, M. J., Illot, M. C., and Peterson, O. H. (1987). J. Membr.
J. Physiol. 246, R985–993.
Biol. 99, 215–224.
34. Hedeskov, C. J. (1980). Physiol. Rev. 60, 442–509.
7. Gembal, M., Detimary, P., Gilon, P., Gao, Z. Y., and Henquin,
35. Malaisse, W. J. (1994). Diabetologia 37 (Suppl. 2), S36–42.
J. C. (1993). J. Clin. Invest. 91, 871–880.
36. Malaisse, W. J., Sener, A., Herchuelz, A., and Hutton, J. C.
8. Sato, Y. and Henquin, J. C. (1998). Diabetes 47, 1713–1721.
(1979). Metabolism 28, 373–386.
9. Lustig, R. H. (2003). Rev. Endocr. Metab. Disord. 4, 23–32.
37. Deeney, J. T., Prentki, M., and Corkey, B. E. (2000). Semin.
10. Ahren, B. (2000). Diabetologia 43, 393–410.
Cell Dev. Biol. 11, 267–275.
11. Tsuura, Y., Ishida, H., Okamoto, Y., et al. (1993). Diabetes 42,
38. Malaisse, W. J., Herchuelz, A., Devis, G., et al. (1978). Ann. NY
Acad. Sci. 307, 562–582.
12. Fournier, L., Begin-Heick, N., Whitfield, J. F., and Schwartz,
39. Kukuljan, M., Li, M. Y., and Atwater, I. (1990). FEBS Lett.
J. L. (1992). J. Membr. Biol. 129, 267–276.
266, 105–108.
13. Sato, Y., Aizawa, T., Komatsu, M., Okada, N., and Yamada, T.
40. Meissner, H. P., Henquin, J. C., and Preissler, M. (1978). FEBS
(1992). Diabetes 41, 438–443.
Lett. 94, 87–89.
14. Straub, S. G., James, R. F., Dunne, M. J., and Sharp, G. W.
41. Rosario, L. M., Atwater, I., and Rojas, E. (1985). Q. J. Exp.
(1998). Diabetes 47, 758–763.
Physiol. 70, 137–150.
15. Westerlund, J., Ortsater, H., Palm, F., Sundsten, T., and Bergsten,
42. Gembal, M., Gilon, P., and Henquin, J. C. (1992). J. Clin.
P. (2001). Eur. J. Endocrinol. 144, 667–675.
Invest. 89, 1288–1295.
16. Chan, C. B. and MacPhail, R. M. (1996). Biochem. Cell Biol.
43. Dunne, M. J., Cosgrove, K. E., Shepherd, R. M., Aynsley-
74, 403–410.
Green, A., and Lindley, K. J. (2004). Physiol. Rev. 84, 239–
17. Abdel-Halim, S. M., Guenifi, A., Khan, A., et al. (1996).
Diabetes 45, 934–940.
44. Gloyn, A. L., Noordam, K., Willemsen, M. A., et al. (2003).
18. Hughes, S. J., Faehling, M., Thorneley, C. W., Proks, P.,
Diabetes 52, 2433–2440.
Ashcroft, F. M., and Smith, P. A. (1998). Diabetes 47, 73–81.
45. Gravena, C., Mathias, P. C., and Ashcroft, S. J. (2002). J. Endo-
19. Balbo, S. L., Mathias, P. C., Bonfleur, M. L., et al. (2000). Res.
crinol. 173, 73–80.
Commun. Mol. Pathol. Pharmacol. 108, 291–296.
46. Moura, A. S., Carpinelli, A. R., Barbosa, F. B., Gravena, C.,
20. Balbo, S. L., Bonfleur, M. L., Carneiro, E. M., Amaral, M. E.,
and Mathias, P. C. (1996). Res. Commun. Mol. Pathol. Phar-
Filiputti, E., and Mathias, P. C. (2002). Diabetes Metab. 28,
macol. 92, 73–84.
3S13–17; discussion 13S108–112.
47. Blair, S. C., Caterson, I. D., and Cooney, G. J. (1993). Diabetes
21. Olney, J. W. (1969). Science 164, 719–721.
42, 1153–1158.
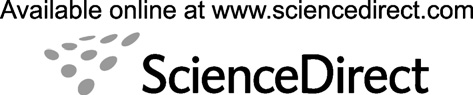
European Journal of Pharmacology 556 (2007) 223 – 228
Muscarinic M2 receptor is active on pancreatic islets from
hypothalamic obese rat
Sabrina Grassiolli, Clarice Gravena, Paulo Cezar de Freitas Mathias ⁎
Laboratory of Secretion Cell Biology, Department of Cell Biology and Genetics, State University of Maringá, Avenida Colombo 5790, 87020-900 Maringá PR Brazil
Received 25 July 2006; received in revised form 6 November 2006; accepted 8 November 2006
Available online 14 November 2006
Hypothalamic obese rats, obtained by neonatal treatment with monosodium L-glutamate (MSG), are hyperinsulinemic, and secrete more
insulin than lean ones do when stimulated by glucose, while acetylcholine insulinotropic effect decreases. The effect of acetylcholine on glucose-induced insulin secretion is attributed to muscarinic receptors of pancreatic beta cells, mainly to M3 subtype. However, it has been observed thatactivation of M2 or M4 subtypes causes inhibition of glucose-induced insulin secretion in insulin secreting cell line. Insulin secretion wasmeasured, stimulated by glucose in the presence of acetylcholine plus methoctramine, a muscarinic M2 antagonist, on pancreatic islets isolatedfrom MSG-obese and lean rats to investigate whether impairment of acetylcholine insulinotropic effect on pancreatic islets from MSG-obese ratshas any relationship with muscarinic M2 receptor function in beta cells. Insulin secretion stimulated by 8.3 mM glucose was higher in islets fromobese rats than from lean ones. Insulinotropic effect of acetylcholine was reported in islets of both animals, albeit less than in obese ones.
Blockage of muscarinic M2 receptor, using methoctramine at 1; 5 and 10 μM, increased acetylcholine secretory response in islets of obese rats,while no effect has been observed in lean ones. Results demonstrate that muscarinic M2 receptors are functioning in pancreatic islets of MSG-obese rats. The inhibitory action of muscarinic M2 receptor may be a mechanism by which acetylcholine discloses weak insulinotropic effect inMSG-obese rats.
2006 Elsevier B.V. All rights reserved.
Keywords: MSG-obese rats; Acetylcholine; Muscarinic M2 receptors; Insulin secretion; Methoctramine; Pancreatic islets
increase in cellular metabolism and ATP production. PotassiumATP sensitive channels (KATP) are inactivated by increased
Peripheral insulin resistance is found both in human and in
ATP/ADP ratio, which provokes membrane depolarization and
several experimental obese animals. It is correlated with high
subsequently activation of Ca2+channels—voltage dependent.
tissue fat accumulation and fasting hyperinsulinemia (
These ionic changes increase intracellular calcium concentra-
tion. High cytosolic free calcium concentration is an intracel-
Hyperinsulinemia may also be a consequence of adaptations on
lular signal that triggers insulin secretion events, accepted as a
pancreatic beta cells. It has been observed that pancreatic islets
triggering pathway that exports insulin to the blood stream.
from obese humans and animals release high amounts of
However, experimental evidences indicate that glucose may be
insulin, stimulated by glucose (
also stimulating insulin secretion by alternative pathways
). Pancreatic beta cells
towards KATP channels In addition, insulin
also secrete insulin when stimulated by different nutrients, such
release is modulated by non nutrient secretagogues, such as
as amino acids, fatty acids, and their metabolites, which induce
neurotransmitters, which enhance or inhibit glucose-stimulatedinsulin secretion. Beta cells are equipped with several receptorsto neurotransmitters and neuropeptides, such as adrenoceptors
and cholinergic receptors. Receptors are stimulated by efferent
Corresponding author. Tel./fax: +55 44 32614892.
E-mail address: (P.C. de Freitas Mathias).
signals from the central nervous system, which include
0014-2999/$ - see front matter 2006 Elsevier B.V. All rights reserved.
S. Grassiolli et al. / European Journal of Pharmacology 556 (2007) 223–228
autonomic nervous system, throughout their neural ends for
to 19:00 h) and temperature (21 ± 2 °C). Water and standard
pancreatic beta cells. During glucose blood level oscillations,
rodent chow (Nuvital-Curitiba-Brazil) were supplied ad libitum.
beta cells receive inputs from the parasympathetic and
When 90 days old, rats from both groups were anesthetized with
sympathetic systems to aid glycemia regulation. Overall
ketamine and xylasine (55 and 8 mg/kg body weight,
acetylcholine promotes potentiation of glucose-induced insulin
respectively) and slaughtered by cervical dislocation. Retroper-
secretion, whereas noradrenaline and adrenaline inhibit it
itoneal fat pads were removed, washed and weighed to estimate
(). Before and during food intake vagus nerve
obesity induced by MSG treatment. Lee index [body weight
activity is responsible for the rise of insulin blood levels. These
(g)1/3/nasal–anal length (cm) × 1000], employed as a predictor
effects are mediated by acetylcholine which activates the
of obesity in MSG-rodents, was calculated.
muscarinic receptor in the beta cell plasma membrane. Fivemuscarinic receptors family (M –
1 M5) have been identified in
2.2. Intravenous glucose tolerance test
neurons and other cell types (). Using RT-PCR technique and different muscarinic
Under anesthesia, previously described, a silicone cannula
antagonists, up to date, 4 muscarinic subtypes (M –
was implanted into the left jugular vein of animals from the two
been shown in insulin-secreting cell line (),
groups and stabilized on the dorsal region of the neck. The
while other studies have demonstrated that 2 muscarinic
cannula was previously treated with heparinized saline (50 IU
subtypes, M1, M3, and in a lesser quantity M5, were found in
heparin/ml 0.9%) to avoid blood clots. After a 12 h fast (19:00–
rat pancreatic islets (). Islets contain 4
07:00 h) and without anesthesia, a glucose load (1 g/kg body
different endocrine cell types, although care must be taken when
weight) was infused in the animals through the cannula.
dealing with muscarinic receptor subtypes of pancreatic beta
Previous to glucose load (5 min), acetylcholine (Ach, 27 nM/kg
cells. Functional studies of muscarinic receptor subtypes have
body weight) was intraperitoneally injected in animals of each
revealed that the M1 and mostly M3 are the receptors involved
group. Blood samples were collected immediately before
in the insulinotropic effect of acetylcholine. Atropine, a non
glucose load (zero time) and at 2, 5, 10, 15, 20, 25 and
specific muscarinic antagonist, reduces hyperinsulinemia in
30 min. Plasma was stored at −20 °C for posterior dosage of
obese humans and rodents
blood glucose concentration by glucose oxidase method
and of insulin by radioimmunoassay (
obese rats, obtained by neonatal treatment with monosodium L-
glutamate (MSG), are hyperinsulinemic (). Asin other experimental obese animals, pancreatic islets isolated
2.3. Insulin tolerance test
from MSG-obese rats oversecrete insulin stimulated by glucose(). However, carbamylcholine, a
Another rat batch, MSG-treated and untreated, fasted
cholinergic agonist, shows less insulinotropic effect on islets
overnight, underwent an intraperitoneal insulin tolerance test
of obese rats than on those of lean ones ),
(1 U/kg body weight of insulin), and samples for blood glucose
unlike islets from other obese rodents, such as ob/ob mice and
measurements were collected at 0 (basal), 2, 5, 10, 15, 20, 25
Zucker rats ). It has
and 30 min after injection. Thereafter, the rate constant for
recently been reported that muscarinic M2 and M4 receptors,
plasma glucose disappearance (Kitt) was calculated by formula
activated by acetylcholine, decrease glucose-stimulated insulin
0.693/(t1/2), as indicated in previous report. The plasma
secretion on insulin-secreting cell line, BRIN-BD 11 (
glucose t1/2 was calculated from the slope of the least square
These surprising cholinergic effects on glucose-
analysis of the plasma glucose concentrations during the linear
induced insulin secretion suggest that changes on quantities
phase of decline (
and/or sensitivities, or alternatively composition of muscarinicreceptors subtypes would participate in cholinergic effects on
2.4. Pancreatic islets isolation
beta cells. The aim of current research is to investigate whetherreduced response to acetylcholine in pancreatic islets of MSG-
Isolation of islets from rat pancreas was performed as
obese rats may be attributed to changes in the function of
previously described ), with adaptations.
muscarinic M2 receptor subtype.
Intact rats, from both groups, were deeply anesthetized, asalready described; the abdominal wall was cut and open. A
2. Materials and methods
10 ml Hank's buffered saline solution (HBSS) containingcollagenase type XI (0.7 mg/ml, Sigma Chemical CO., St.
2.1. Animals and obesity
Louis, MO) was injected into the rat's common bile duct. Thepancreas, swollen with the collagenase solution, was quickly
Neonate male Wistar rats were subcutaneously injected,
excised and incubated in a plastic culture bottle for 15 min at
during the first 5 days of life, with monosodium L-glutamate
37 °C. Suspension was then filtered with a 0.5 mm metal mesh
(MSG), at a dose of 4 g/kg body weight. Control animals
and washed with HBSS, containing 0.12% bovine serum
received equimolar saline solution. Both animal groups were
albumin fraction V (BSA), in 5 continuous washings. Islets
weaned when 21 days old. All animals were housed, under
were collected with the aid of a microscope. At least 6 rats were
control conditions, in a 12-h light–dark cycle luminosity (07:00
used to obtain a pancreatic islet pool for each animal group.
S. Grassiolli et al. / European Journal of Pharmacology 556 (2007) 223–228
2.5. Islets incubation
to untreated rats, p b 0.001. Indeed, MSG-rats accumulated 2.85fold in fat deposit of retroperitoneal pad than controls, as shown
Batches of 4 islets were pre-incubated for 60 min in 1 ml of
in , p b 0.0001. MSG-rats present an increase of 200%
normal Krebs–Ringer solution containing: 120 mM NaCl;
fasting insulin blood levels, p b 0.0001, while glucose blood
4.8 mM KCl; 2.5 mM CaCl2; 1.2 mM MgCl2; 24 mM NaHCO3
concentration was unchanged when compared to that of control
and 5.6 mM glucose. This solution was gassed O2/CO2 (95/5%)
rats (MSG-animals were insulin resistant when
to maintain pH 7.4 and supplemented with BSA (0.12%). After
compared to control rats, as expressed by their lower plasma
adaptation to a low glucose concentration (5.6 mM) solution,
glucose disappearance rates measured by the insulin tolerance
islets underwent incubations for a further 60 min in glucose
test. The latter showed a reduction of glucose disappearance
8.3 mM with Krebs–Ringer solutions. Islets were also
rates (Kitt) up to 36%, p b 0.001, as shown in
incubated with acetylcholine at different concentrations: 0.1;1.0; 10; 100 and 1000 μM in Krebs–Ringer solution with
3.2. Glucose tolerance test and exogenous acetylcholine effects
glucose 8.3 mM. The degradation of acetylcholine was avoidedby neostigmine (10 μM), an inhibitor of acetylcholinesterase
A shows that, after glucose load, MSG-rats have
enhanced glucose blood levels by 2.5 fold throughout glucosetolerance test when compared to controls, p b 0.001. Blood
2.6. Effect of muscarinic M2 antagonist
insulin rose 70% in MSG-rats at 2–20 min after glucoseinfusion when compared to controls in the same period,
After adaptation to a low glucose concentration solution,
p b 0.001, as shown in B. Pre-treatment of acetylcholine
islets underwent incubations for a further 60 min in glucose
in control rats induced a 21% reduction of plasma glucose at 2;
8.3 mM with Krebs–Ringer solutions with acetylcholine
5; and 10 min after glucose load, p b 0.05 (C). In these
(10 μM) and neostigmine (10 μM) at different concentrations,
conditions, plasma insulin increased by 54% at 2 and 5 min,
1; 5 and 10 μM of muscarinic antagonist to subtype M2,
p b 0.05, as shown in However, exogenous acetylcho-
methoctramine. Aliquots of all incubations were used by
line in MSG-rats failed to change plasma insulin and glucose
radioimmunoassay insulin dosage.
throughout the entire ivGTT when results, obtained without pre-treatment with cholinergic agent, are compared, as E and
Methoctramine tetrahydrochloride, monosodium L-gluta-
3.3. Glucose-stimulated insulin secretion on isolated pancrea-
mate and human insulin standard came from Sigma Chemical
Co. (St Louis MO). Human Recombinant Insulin (Humalog)was purchased from Eli Lilly (Indianapolis, IN). [I125] Human
In A, glucose 8.3 mM stimulated 2 fold insulin release
insulin was acquired from Pharmacia (São Paulo, BR). Routine
in islets of MSG-rats when compared to islets of control rats,
reagents were purchased from Sigma, unless otherwise
3.4. Dose-response of acetylcholine insulinotropic effect
2.8. Data analysis
Cholinergic agent enhanced in a dose-dependent manner the
Results are given as mean ± S.E.M. Data were submitted to
glucose-induced insulin release to 79, 170, 290, 295 and 300%,
Student's t-test or variance analysis (ANOVA). In the case of
respectively, with acetylcholine concentrations of 0.1; 1; 10;
analyses with a significant F, the differences between means
100 and 1000 μM, in control rats' islets, p b 0.01. However,
were evaluated by Bonferroni t-test. P values less than 0.05
islets of MSG-rats responded less to acetylcholine: 0, 20, 53, 35
were considered statistically significant. The tests were
and 39% at similar concentrations when used in islets of control
performed using GraphPad Prism version 3.02 for Windows
animals, p b 0.05, as shown in B.
The Ethical Committee for Animal Experiments of the State
University of Maringá, Maringá PR Brazil approved the
Effects of MSG-neonatal treatment in adult rats
0.338 ± 0.002 ⁎
Retroperitoneal fat (g/100 g bw)
Basal insulin (ng/mL)
3.1. Effects of MSG neonatal treatment on fat accumulation,
Basal glycemia (mmol/L)
fasting plasma insulin and glucose and peripheral insulin
resistance of adult rats
Data represent mean ± S.E.M. obtained from 10–20 animals in the two groups ofanimals. Lee index and Kitt, as described in methods, were calculated. Plasmainsulin and glucose concentration were obtained after overnight fasting.
Treatment with MSG of suckling pups induced obesity in
Student's t-test was used to analyze differences between MSG and control rats.
adult rats, as indicated by a Lee index rise of 8% when compared
S. Grassiolli et al. / European Journal of Pharmacology 556 (2007) 223–228
high lipogenesis (Owing to these results, itmay be suggested that the high sensitivity on white adipose tissue,counterbalances on a long term the insulin resistance in othertarget tissues. Such mechanism causes MSG-obese rats tomaintain normal fasting plasma glucose and prevent developmentof type 2 diabetes. Results indicate that, in fed state, imitated byglucose tolerance test, MSG-obese rats present glucose intoler-ance attributed to insulin resistance. Inducing insulin blood levelrise, vagus nerve activity has been shown to participate inglycemia control in pre- and post-prandial states It has been demonstrated that exogenous acetylcholine hasan insulinotropic effect in lean rats, during glucose tolerance test,as reported by other authors ). However, the cholinergic agent had no effect oninsulin and glucose concentrations of MSG-obese rats' plasma. Inobese humans and rodents, infusion of cholinergic agents inducedhigher insulin secretion than in control lean ones These conflicting results may have originated by differentmechanisms of control insulin secretion displayed on beta cells.
It is very interesting that mice with super expression of muscarinicM3 receptor gene are more resistant to glucose intolerance thanwide mice. In fact, the transgenic animal secretes high amounts of
Fig. 1. Glucose tolerance test and effect of exogenous acetylcholine. Eachsymbol in the figures represents mean obtained from 8–10 rats in two groups ofrats. Increment of plasma glycemia is shown on right panels (A, C, E) andplasma insulin on left panels (B, D, F), with or without acetylcholine (27 nM/kgbody weight). Lines over symbols represent S.E.M. Student's t-test was used toanalyze differences between MSG-treated rats and control rats. ⁎p b 0.05.
3.5. Effect of muscarinic M2 antagonist, methoctramine, oninsulin secretion potentiated by acetylcholine
shows that insulinotropic effect of acetylcholine was
unchanged by methoctramine in islets of control rats.
Surprisingly, methoctramine enhanced 3 fold the insulinotropiceffect of acetylcholine in islets of MSG-rats, p b 0.001, whereasthis effect was identical in three methoctramine concentrations.
Results confirm that MSG-treated neonate rodents develop
obesity in adult life, and they present insulin resistance and highlevels of fasting plasma insulin while without hyperglycemia().
Neurons of hypothalamic arcuate nucleus are destroyed by MSGtreatment in rats' suckling pups The experimentalanimal model of MSG-obesity presents different characteristics,when compared to other obese rodents, such as those geneticallyoriginated (ob/ob mice and Zucker rats) or those with
Fig. 2. Insulin secretion stimulated by glucose 8.3 mM and acetylcholine. In Fig.
hypothalamic lesion. Unlike other experimental obesity up to
2A islets of the two groups were incubated for 60 min in presence of glucose
210 days of life, MSG-rats do not develop hyperglycemia, even
8.3 mM. Each bar represents mean of 20 observations. In Fig. 2B islets were
though target tissues to insulin action present an unusual behavior.
incubated in medium containing glucose 8.3 mM plus acetylcholine at differentconcentrations: 0.1; 1; 10; 100 and 1000 μM; and neostigmine 10 μM. Each
While liver and muscle tissues show installed insulin resistance,
symbol represents mean of 20 observations on the two islet groups. Lines over
white adipose tissue fails to have any resistance at all
symbols represent S.E.M. Student's t-test was used to analyze differences
). Instead, white adipose tissue develops insulin-dependent
between MSG-treated rats and control rats. ⁎p b 0.05.
S. Grassiolli et al. / European Journal of Pharmacology 556 (2007) 223–228
Fig. 3. Effects of muscarinic subtype M2 antagonist on glucose-induced insulin secretion potentiated by acetylcholine. Bars represent mean obtained from 20observations from each islet groups. Lines over bars represent S.E.M. All islets batches were incubated in medium containing glucose 8.3 mM in the presence ofneostigmine 10 μM. As indicated, acetylcholine and/or muscarinic M2 antagonist methoctramine in 3 different concentrations were added to incubations. Asterisksover bars represent statistical differences tested by ANOVA: ⁎ – glucose 8.3 mM; ⁎ ⁎ – glucose 8.3 mM + acetylcholine (10 μM); ⁎ ⁎ ⁎ – glucose 8.3 mM +
methoctramine (1 μM); ⁎ ⁎ ⁎ ⁎ – glucose 8.3 mM + acetylcholine (10 μM) + methoctramine (1 μM); ⁎ ⁎ ⁎ ⁎ ⁎ – glucose 8.3 mM + acetylcholine (10 μM) +methoctramine (5 μM) and ⁎ ⁎ ⁎ ⁎ ⁎ ⁎ – glucose 8.3 mM + acetylcholine (10 μM) + methoctramine (10 μM). Fig. 3 A represents data from islets isolated from control
rats, whereas Fig. 3 B shows islets from MSG-obese rats.
insulin during glucose tolerance test when compared to the wide
vivo and in vitro, may be a consequence of the reorganization of
type (Pancreatic islets from MSG-obese rats
muscarinic receptor subtypes composition on beta cell plasma
secrete higher insulin quantities than lean controls, a fact already
membrane in response to obesity onset. In other cell types with
observed in other obese rodents
different muscarinic receptor subtypes, acetylcholine induced M2
Regardless of the high
biding-caused inhibitory effects (Other studies
cholinergic insulinotropic effect on islets isolated from other
have also shown that high concentration of cholinergic agents
obese rodents, islets of MSG-obese rats showed low responsivity
inhibit the glucose-stimulated insulin secretion
to acetylcholine. It has been shown that MSG-rodents have high
and produce an early transient hyperpolarization of beta
vagal activity, including efferents to the endocrine pancreas
cells when islets are perifused with a stimulating concentration of
glucose (). Therefore,
reported in many other obese rodents
protein and genic characterization of muscarinic M2 receptor, as
). Results with islets isolated from MSG-obese indicate that
well as functional studies, must be conducted to elucidate whether
alterated control of insulin secretion is a result of adaptations,
muscarinic M2 receptor plays a role in the insulin secretion control
which allow beta cells to better respond to glucose-induced
both in normal and pathological states.
insulin secretion and impair cholinergic insulinotropic effects.
Current authors would like to conclude that results show that
The first evidence that acetylcholine may inhibit the glucose-
hyperinsulinemia of obese adult rat, obtained by MSG neonatal
stimulated insulin secretion has been demonstrated in insulin
treatment, is related to enhancement of glucose-stimulated insulin
secreting cell line, using pharmacological agents, which blocked
secretion. Besides its classical insulinotropic effects, acetylcho-
muscarinic M2 receptors (However, this
line displayed an inhibitory action in obese rats, which may be
effect has not been found in islets of normal rats, even when the
attributed to the function of muscarinic M2 receptor observed on
same antagonist, methoctramine, at the same concentration, was
pancreatic islets. Current findings may have clinical significance
used, as other authors have reported. It has to be emphasized that
for the control of insulin secretion in metabolic disturbances, such
insulin secreting cell lines have a different control of insulin
as obesity and diabetes.
secretion when compared to pancreatic islets' beta cellsUnexpectedly, islets of MSG-
obese rats showed a clear response to pharmacological blockageof muscarinic M2 receptor. Although methoctramine seems to be
We gratefully acknowledge the financial support of CNPq
also an antagonist to muscarinic M4 receptor, it presents higher
(Brazilian Council for Scientific and Technological Development).
pharmacological effects on muscarinic M2 receptor than on M4subtype ). In insulin secreting cell line BRIND
BD11 muscarinic M4 receptor is also involved in the inhibition ofglucose-induced insulin secretion ). Results
Ahren, B., 2000. Autonomic regulation of islet hormone secretion—implica-
tions for health and disease. Diabetologia 43, 393410.
may indicate that, at least partially, islets of MSG-obese have
Ahren, B., Sauerberg, P., Thomsen, C., 1999. Increased insulin secretion and
functional muscarinic M2 receptor activity. Lower acetylcholine
normalization of glucose tolerance by cholinergic agonism in high fat-fed
insulinotropic effects displayed in MSG-obese rats, observed in
mice. Am. J. Physiol. 277, E93E102.
S. Grassiolli et al. / European Journal of Pharmacology 556 (2007) 223–228
Atef, N., Brule, C., Bihoreau, M.T., Ktorza, A., Picon, L., Penicaud, L., 1991.
downstream signaling in insulin sensitive tissues of MSG-rats. Life Sci. 73,
Enhanced insulin secretory response to acetylcholine by perifused pancreas
of 5-day-old preobese Zucker rats. Endocrinology 129, 22192224.
Iismaa, T.P., Kerr, E.A., Wilson, J.R., Carpenter, L., Sims, N., Biden, T.J., 2000.
Balbo, S.L., Bonfleur, M.L., Carneiro, E.M., Amaral, M.E., Filiputti, E.,
Quantitative and functional characterization of muscarinic receptor subtypes
Mathias, P.C., 2002. Parasympathetic activity changes insulin response to
in insulin-secreting cell lines and rat pancreatic islets. Diabetes 49, 392398.
glucose and neurotransmitters. Diabetes. Metab. 28, 3S133S17 discussion
Jeanrenaud, B., 1983. Energy fuel and hormonal profile in experimental
obesities. Experientia, Suppl. 44, 5776.
Benthem, L., Mundinger, T.O., Taborsky Jr., G.J., 2000. Meal-induced insulin
Leon-Quinto, T., Magnan, C., Portha, B., 1998. Altered activity of the
secretion in dogs is mediated by both branches of the autonomic nervous
autonomous nervous system as a determinant of the impaired beta-cell
system. Am. J. Physiol.: Endocrinol. Metab. 278, E603E610.
secretory response after protein-energy restriction in the rat. Endocrinology
Bergmeyer, H.B.E., 1974. Determination of glucose with glucose oxidase and
139, 33823389.
peroxidase. Methods of Enzymatic Analysis, pp. 11051212.
Bonner, T.I., 1989. The molecular basis of muscarinic receptor diversity. Trends
secretion and acetylcholinesterase activity in monosodium L-glutamate-
Neurosci. 12, 148151.
induced obese mice. Horm. Res. 54, 186191.
Bonner, T.I., Young, A.C., Brann, M.R., Buckley, N.J., 1988. Cloning and
Macho, L., Fickova, M., Jezova, Zorad, S., 2000. Late effects of postnatal
expression of the human and rat M5 muscarinic acetylcholine receptor
administration of monosodium glutamate on insulin action in adult rats.
genes. Neuron 1, 403410.
Physiol. Res. 49 (Suppl 1), S79S85.
Bordin, S., Boschero, A.C., Carneiro, E.M., Atwater, I., 1995. Ionic mechanisms
Marçal, A.C., Grassiolli, S., Rocha, D.N., Puzzi, M.A., Gravena, C., Scomparin,
involved in the regulation of insulin secretion by muscarinic agonists.
D.X., et al., in press. The dual effect of isoproterenol on insulin release is
J. Membr. Biol. 148, 177184.
suppressed in pancreatic islets from hypothalamic obese rats. Endocrine 29.
Bray, G.A., 2004. Medical consequences of obesity. J. Clin. Endocrinol. Metab.
Marmo, M.R., Dolnikoff, M.S., Kettelhut, I.C., Matsushita, D.M., Hell, N.S.,
89, 25832589.
Lima, F.B., 1994. Neonatal monosodium glutamate treatment increases
Campfield, L.A., Smith, F.J., 1983. Neural control of insulin secretion:
epididymal adipose tissue sensitivity to insulin in three-month-old rats. Braz.
interaction of norepinephrine and acetylcholine. Am. J. Physiol. 244,
J. Med. Biol. Res. 27, 12491253.
McClenaghan, N.H., Flatt, P.R., 1999. Engineering cultured insulin-secreting
Caulfield, M.P., 1993. Muscarinic receptors—characterization, coupling and
pancreatic B-cell lines. J. Mol. Med. 77, 235243.
function. Pharmacol. Ther. 58, 319379.
Miguel, J.C., Abdel-Wahab, Y.H., Mathias, P.C., Flatt, P.R., 2002. Muscarinic
Chan, C.B., 1993. Glucokinase activity in isolated islets from obese fa/fa Zucker
receptor subtypes mediate stimulatory and paradoxical inhibitory effects on
rats. Biochem. J. 295 (Pt 3), 673677.
an insulin-secreting beta cell line. Biochim. Biophys. Acta 1569, 4550.
Chan, C.B., MacPhail, R.M., Mitton, K., 1993. Evidence for defective glucose
Olney, J.W., 1969. Brain lesions, obesity and other disturbances in mice treated
sensing by islets of fa/fa obese Zucker rats. Can. J. Physiol. Pharmacol. 71,
with monosodium glutamate. Science 164, 719721.
Renuka, T.R., Robinson, R., Paulose, C.S., 2006. Increased insulin secretion by
Del Rio, G., Procopio, M., Bondi, M., Marrama, P., Menozzi, R., Oleandri, S.E.,
muscarinic M1 and M3 receptor function from rat pancreatic islets in vitro.
et al., 1997. Cholinergic enhancement by pyridostigmine increases the
Neurochem. Res. 31.
insulin response to glucose load in obese patients but not in normal subjects.
Rohner-Jeanrenaud, F., Hochstrasser, A.C., Jeanrenaud, B., 1983a. Hyperinsu-
Int. J. Obes. Relat. Metab. Disord. 21, 11111114.
linemia of preobese and obese fa/fa rats is partly vagus nerve mediated. Am.
Eglen, R.M., Reddy, H., Watson, N., Challiss, R.A., 1994. Muscarinic
J. Physiol. 244, E317E322.
acetylcholine receptor subtypes in smooth muscle. Trends Pharmacol. Sci.
Rohner-Jeanrenaud, F., Ionescu, E., Jeanrenaud, B., 1983b. The origins and role
15, 114119.
of efferent vagal nuclei in hyperinsulinemia in hypothalamic and genetically
Fukudo, S., Virnelli, S., Kuhn, C.M., Cochrane, C., Feinglos, M.N., Surwit, R.S.,
obese rodents. J. Auton. Nerv. Syst. 9, 173184.
1989. Muscarinic stimulation and antagonism and glucoregulation in
Santos, R.M., Rojas, E., 1989. Muscarinic receptor modulation of glucose-
nondiabetic and obese hyperglycemic mice. Diabetes 38, 14331438.
induced electrical activity in mouse pancreatic B-cells. FEBS Lett. 249,
Gautam, D., Han, S.J., Hamdan, F.F., Jeon, J., Li, B., Li, J.H., et al., 2006. A critical
role for beta cell M3 muscarinic acetylcholine receptors in regulating insulin
Scott, A.M., Atwater, I., Rojas, E., 1981. A method for the simultaneous
release and blood glucose homeostasis in vivo. Cell. Metab. 3, 449461.
measurement of insulin release and B cell membrane potential in single
Gravena, C., Mathias, P.C., Ashcroft, S.J., 2002. Acute effects of fatty acids on
mouse islets of Langerhans. Diabetologia 21, 470475.
insulin secretion from rat and human islets of Langerhans. J. Endocrinol.
Tassava, T.M., Okuda, T., Romsos, D.R., 1992. Insulin secretion from ob/ob
173, 7380.
mouse pancreatic islets: effects of neurotransmitters. Am. J. Physiol. 262,
Guldstrand, M., Ahren, B., Adamson, U., 2003. Improved beta-cell function
after standardized weight reduction in severely obese subjects. Am. J.
Teff, K.L., Townsend, R.R., 1999. Early phase insulin infusion and muscarinic
Physiol.: Endocrinol. Metab. 284, E557E565.
blockade in obese and lean subjects. Am. J. Physiol. 277, R198R208.
Henquin, J.C., 2000. Triggering and amplifying pathways of regulation of
Thirone, A.C., Carvalheira, J.B., Hirata, A.E., Velloso, L.A., Saad, M.J., 2004.
insulin secretion by glucose. Diabetes 49, 17511760.
Regulation of Cbl-associated protein/Cbl pathway in muscle and adipose
Hirata, A.E., Alvarez-Rojas, F., Carvalheira, J.B., Carvalho, C.R., Dolnikoff,
tissues of two animal models of insulin resistance. Endocrinology 145,
M.S., Abdalla Saad, M.J., 2003. Modulation of IR/PTP1B interaction and
Obesity onset in MSG-rat induces progressive impairment of acetylcholine
insulinotropic effect on pancreatic islets
Sabrina Grassiolli, Sandra Lucinei Balbo, Ana Eliza Andreazzi, Rosana Torrezan,
Clarice Gravena and Paulo Cezar de Freitas Mathias*.
Laboratory of Secretion Cell Biology, Department of Cell Biology and Genetics, State
University of Maringá, 87020-900 Maringá PR Brazil.
*Address for correspondence
Paulo Cezar de Freitas Mathias
Laboratory of Secretion Cell Biology
Department of Cell Biology and Genetics
State University of Maringá
Avenida Colombo 5790
87020-900 Maringá PR BRAZIL
Phone/fax: 00 55 44 32614892
E-mail: [email protected]
Key words: obesity, MSG, acetylcholine, pancreatic islets, insulin secretion
Running title: Acetylcholine insulinotropic effect in MSG-obesity onset
Abstract
Different from other obese animals, hyperinsulinemic adult obese MSG-rats do not show
hyperglycemia. Insulin oversecretion stimulated by high glucose concentration and low
response to cholinergic agents in pancreatic islets from MSG-obese adult rat has been
documented. Current study questions whether changes on insulin secretory response to
glucose and acetylcholine occur during post-weaning development of rats or whether the
latter is modified by MSG-obesity onset. Fasting MSG-treated and untreated rats were
sacrificed after 30, 60 and 90 days of birth. Blood samples were used to measure glucose
and insulin levels. Periepididymal fat pads were taken to estimate body fatness. Isolated
pancreatic islets were incubated for 60 min in the presence of glucose concentration (3.9-
28.8 mM), whereas other batches of islets were incubated with 8.3 mM glucose in the
presence of acetylcholine (0.01-3000 µM). Samples from incubation media were collected
to measure insulin release. Although development provoked progressive high fat
accumulation; it was higher in MSG-treated rats. Rises in body fatness were followed by
insulin increase in both groups, MSG-obese rats presented hyperinsulinemia. Regardless of
development and treatment, fasting normoglycemia was maintained. Development caused a
progressive slight decrease in maximum secretory response induced by glucose in islets
from lean rats, while glucose response was maintained in islets from MSG-obese rats.
Development did not alter glucose sensitivity (15 mM). MSG treatment caused only
sensitivity increase to glucose in islets from 60-day-old rats. While development did not
change the acetylcholine insulinotropic response, islets from MSG-treated rats showed
progressive decrease in the same response. Islets from all ratgroups failed to alter
acetylcholine sensitivity. Results suggest that MSG treatment accelerates adaptations on
beta cells which occur during post-weaning development. However, changes did not cause
enough perturbation on beta cells to induce hyperglycemia onset.
Hyperinsulinemia, glucose intolerance and peripheral insulin resistance are main hallmarks
of human and experimental obese animals which frequently develop hyperglycemia and,
consequently, diabetes (1, 2). Therefore, obesity onset has a phase in which glucose, even
at low concentration, stimulates high insulin secretion and another one in which glucose,
even in high concentration, is not able to cause a necessary insulin secretion to guarantee
enough glucose uptake by tissues and surmount insulin resistance. In the later the
pancreatic beta cells lose their capacity to secrete insulin with regard to blood glucose level
oscillations. Cellular metabolism is determinant to trigger the insulin secretion process in
beta cells. Metabolism is activated by glucose degradation which, in general, increases
ATP/ADP ratio and potassium ATP sensitive channels (K+ATP channel) are inactivated
provoking beta cell membrane depolarization. Subsequently voltage-sensitive calcium
channels are opened and free cytosolic calcium concentration is increased, which triggers
the exocytosis of insulin secretory vesicles (3, 4). While other pathways independent of
K+ATP channel has been suggested, the beta cell metabolism activation is important to
trigger insulin granules exocytosis (5). Glucose is not the only nutrient secretagogue since
amino-acids and fatty acids, using the same pathways, can also stimulate insulin secretion.
In addition, non-nutrient secretagogues, such as neurotransmitters, among others, enhance
or inhibit glucose-induced insulin secretion so that beta cells may release insulin to improve
control blood glucose levels. Blood insulin concentration is under central nervous system
(CNS) control. Preceding and anticipated food intake vagal activity promotes a transient
insulin secretion, known as cephalic phase. High vagal activity, as an important component
of hyperinsulinemia, has been observed in human and experimental animal obesity.
Vagotomy or atropinization may reduce hyperinsulinemia (6-9). Many neurotransmitter
receptors have been found in the beta cell plasma membrane (10). Muscarinic receptors
activated by acetylcholine, which is released from vagal ends to beta cells, induce cytosolic
calcium concentration rise and enhance glucose insulinotropic response (11). Functional
studies of muscarinic receptor subtypes have revealed that the M1 and mostly M3 are the
receptors involved in the insulinotropic effect of acetylcholine (12, 13).
Perinatal life is important to the development of the whole organism and the
functioning of the pancreatic islets. Any nutritional disturbances or other injuries during
fetus or neonatal life, in human and in laboratory animals, may cause permanent
metabolicalterations, which lead to obesity and diabetes, among other chronic diseases, in
adult life (14-16). Reduced insulin secretion has been reported in pancreatic islets from
neonatal, post-weaning and young rats as a response to high glucose stimulation, when
compared to islets of adult rats (17, 18). A decline in insulin secretory response from
pancreatic islets with aging has also been observed (15, 19, 20). Degenerative diseases
onset with high prevalence worldwide, such as obesity and non-insulin-dependent diabetes
(NIDDM), has been observed in aging population. It has been demonstrated that in 60-year-
old people or over the prevalence of NIDDM increases when compared to that in younger
people (21). Glucose-induced insulin secretion is impaired in obese and diabetic patients,
both young and old. Diabetes may be defined as an imbalance between insulin sensitivity
and beta cell function, either or both may worsen with age. Four decades ago obesity has
been associated with increased insulin secretion (22, 23); it has also been pointed out that
insulin oversecretion in obesity is not caused by the obesity per se but by concomitant
reduced insulin sensitivity. The mechanism underling the islet adaptation to insulin
resistance, or rather, how beta cell may sense a reduction in insulin sensitivity, is not yet
known. Insulin resistance increases in aging rats (24). However, whether alterations in
insulin sensitivity and pancreatic beta cell function exist and progress in the early stage of
development, from the post-weaning phase through adult life, are still unknown.
Aging is also accompanied by changes in cholinergic neurons in both the central
and the peripheral nervous system (25, 26). It has been observed that insulin release is
decreased in response to acetylcholine in islets from aged lean mice, and reduced
sensitivity for cholinergic stimulation in islets of ob/ob aged obese mice (27).
Administrations of monosodium L- glutamate (MSG) to rodents' suckling pups promote
the death of neurons in hypothalamic areas and induce changes in the development of the
CNS. Adult MSG-treated rats and mice exhibit disturbances in body weight control, which
lead to increased adiposity, hyperinsulinemia and insulin resistance. Unlike other obese
rodents, such as those from genetic origin or from lesions in CNS, overeating in MSG-
rodents does not occur. In spite of hyperinsulinemia and insulin resistance, adult MSG-rats
are normoglycemic (9, 28, 29). It is surprising that adult MSG-obese rats do not develop
fasting hyperglycemia and diabetes. While pancreatic islets from these adult obese rats
oversecreted insulin stimulated by high glucose concentration, secretory cholinergic
response is reduced in MSG-obese animals (9). With regard to development, from post-
weaning to adult life and the MSG-obesity onset, no investigations on insulin secretion
stimulated by glucose and cholinergic agents were conducted to date. The aim of current
research is to investigate: i) the glucose-stimulated insulin secretion and acetylcholine
insulinotropic effect on pancreatic islets of rats, from the post-weaning through adult life,
and ii) whether onset of MSG-obesity accelerates changes in the functioning of beta cells.
Materials and Methods.
Animals: Neonate Wistar rats received intradermically during 5 first days of life MSG
injections with a dose of 4g/Kg body weight (BW). Control animals received equimolar
saline solution. Animals from both groups were weaned at 21 days of age. All animals were
housed under controlled cycle luminosity conditions of 12 hours light-dark (07:00 to 19:00
h) and temperature (21±2ºC). Water and standard rodent chow (Nuvital – Curitiba – Brazil)
were supplying ad libitum. Only males were used in experimental protocols. MSG-treated
and control rats were divided into three groups: post-weaned rats (30 days), young (60
days) and adult young rats (90 days) used for all protocols. After a 12h fast, rats from all
groups were anesthetized with ketamine and xylasine (55 and 8 mg/Kg BW, respectively)
and sacrificed by cervical dislocation; samples of total blood were used to measure fasting
plasma glucose and insulin levels, by glucose-oxidase technique (30) and radioimmunoassay
(RIA) (31), respectively. Periepididymal fat pad were removed, washed and weighed to
estimate body fatness.
Pancreatic Islets: Isolation of islets from rat pancreas was performed as previously
described (32), with adaptations. Rats from other samples were deeply anesthetized with
ketamine and xylasine, and the abdominal wall was cut and opened. A 10 ml Hank's
buffered saline solution (HBSS) containing collagenase type V (0.7 mg/ml, Sigma
Chemical CO., St. Louis, MO) was injected into the common bile duct of the rat. The
pancreas, swollen with the collagenase solution, was quickly excised and incubated in a
plastic culture bottle for 15 min at 37ºC. The suspension obtained was filtered with a
0.5mm metal mesh and washed with HBSS, including 0.12% bovine serum albumin
fraction V (BSA) in 5 continuous washings. The islets were collected with the aid of a
microscope. At least 3 rats were used to obtain a pancreatic islets pool for each animal
Islets Incubation: Batches of 4 islets were pre-incubated for 60 min in 1mL normal Krebs
solution containing: 120mM NaCl; 4.8mM KCl; 2.5mM CaCl2; 1.2mM MgCl2; 24mM
NaHCO3 and 5.6 mM glucose. This solution were gassed O2/CO2 (95/5%) to maintain pH
7.4 and supplemented with BSA (0.12%) and used during the next protocols.
Glucose-induced insulin release: After pre-incubation period, islets underwent a further
60min incubation in different glucose concentrations: 3.9; 4.7; 5.6; 6.7; 8.0; 9.6; 11.1; 13.3;
16.7; 20.0; 24.0 and 28.8mM in Krebs solution. Samples of incubation media were taken
and stored frozen until assay for measuring secreted insulin by RIA.
Ach insulinotropic effect: After pre-incubation, other islet batches were incubated for 60 min
in Krebs solution containing: glucose 8.3mM in the presence of acetylcholine in different
concentrations: 0.01; 0.1; 0.3; 1; 3; 10; 30; 100; 300; 1000 and 3000µM. Neostigmine
(10µM), an inhibitor of the acetylcholinesterase activity, was used to avoid acetylcholine
degradation. Samples of incubation media were taken and stored frozen until assay for
measuring secreted insulin by RIA.
Materials: Ach, MSG, neostigmine and standard human insulin human were purchased
from Sigma (St. Louis, MO). [I125] human insulin was obtained for Amershan Pharmacia
(São Paulo, BR). When not specified other routine reagents came from Sigma.
Data analysis: Results were given as mean ± SEM. Data from control groups and MSG-
treated rats of the same age were submitted to Student t test. Variance analysis (ANOVA)
was used to test differences between groups at different ages to control and MSG-rats. In
the case of analyses with a significant F, differences between means were evaluated by
Bonferroni post- test. Dose-response curves were generated through a sigmoid equation
(Response = Min+ (Max-Min)/(1+10(LogEC50-Dose) x Hill Slope))), processed with Graph Pad
Prism version3.02 to Windows (Graph Pad Software, San Diego California, USA).
Differences were considered significant at p<0.05.
MSG obesity onset: Development induced progressive increase in periepididymal fat pad
on MSG treated or on untreated rats. MSG-rats presented two times more periepididymal
fat pad content than control animals in all ages (p<0.05). While MSG-rats increased
periepididymal fat pad content 4 fold for 30 to 90 days of age, in control animals increase
was 3 fold (p<0.05), as shown in Figure 1A. Fasting blood glucose concentrations, shown
in Figure 1B, are unchanged by development in both groups, MSG treated or untreated rats.
Fasting blood insulin levels were significantly enhanced 4 fold for MSG-rats at 90 days old
and 2.5 fold for control rats when compared with the younger ones, respectively, p<0.01, as
shown in Figure 1C. However, neonatal MSG treatment caused rises of 2, 3 and 4 fold on
fasting insulin levels when compared to untreated animals at 30, 60 and 90 days,
respectively, p<0.01.
Glucose-induced insulin secretion: Glucose-stimulated insulin secretion discloses a dose-
dependent curve on pancreatic islets isolated from 30, 60 and 90 days old MSG treated or
untreated rats, as showed in Figures 2A, 2B and 2C, respectively. Islets from 60-day-old
MSG-rat showed a 12% higher sensitivity to glucose, as calculated by EC50, than control
ones, p<0.05, as shown in Table 1. However, glucose sensitivity was not different in 30-
and 90-day-old animals, when tested on MSG and control rats. Table 1 also shows that
development of rats from both groups does not change glucose sensitivity, while 60-day-
old MSG-rats present a glucose sensitivity increase by 7% when compared to 30- and 90-
day-old ones, p<0.05. Using the insulin release values from curves, the glucose maximum
response was calculated. Table 1 shows that MSG treatment caused a 50% increase on
glucose response of islets from 90-day-old rats when compared to control ones at same age,
p<0.05. However, glucose maximum response obtained with islets of 30- and 60-day-old
MSG-rats were 19 and 30% lower than the islets of control ones, respectively, p<0.05.
Table I also shows that islets from 60-day-old control rats presented a decrease by 28% in
glucose maximum response when compared to 30-day-old rats, p<0.05, while the value for
60-day-old rats did not change in islets of 90-day-old control rats. Islets from 30- and 90-
day-old MSG-rats did not show any significant difference; however at 60 days of age
glucose maximum response decrease by 50%, p<0.05, as shown also in Table I.
Ach insulinotropic effect: Figure 2 shows that presence of Ach in islets isolated from all
animals at any age, incubated with glucose 8.3 mM, display a dose-dependent curve.
However MSG treatment shifted to right all curves. As shown in Table II, Ach sensitivity,
as calculated by EC50, was still unchangeable, regardless of treatment or age. Ach
maximum response decreases in islets from young control rat (47%) and those from adult
control rats (24%), when compared to weaned rats, p<0.05. MSG treatment progressively
decreased Ach maximum response: 29% at 30, 75% at 60 and 91% at 90 days old, when
compared to control rats at respective ages, p<0.05. Table II also shows that development
of weaned rats treated with MSG caused a progressive decrease in Ach maximum response
on their islets, 74% and 80% to young and adult rats, respectively, p<0.05.
Discussion
An increase in body fatness occurs during development in rodents and humans (13, 33-35).
After weaning up to adult life, control rats showed a clear progressive fat accumulation in
normal rats and an increasing fasting blood insulin concentration. When body fatness rises,
more insulin is secreted to allow glucose to be consumed by tissues, as an adaptation. In
these conditions blood glucose concentration is maintained low, or in the case of an
uncontrolled situation, hyperglycemia is observed (36-38). While, progressive increased
fatness and blood insulin were observed in control rat during early post-weaning
development, fasting glycemia was not changed. Results in current research show that
MSG neonatal treatment exacerbates changes on body fatness and fasting insulin levels,
while maintaining normoglycemia, during development. Our laboratory and others have
demonstrated that MSG treatment during early lactation causes obesity in adult rodents
together with fasting hyperinsulinemia (9, 38-40). Present results indicate that disturbance
in body weight control and insulin secretion start early in MSG-treated rats; however,
development per se promotes changes in the control of body fatness with possible
synchronized adaptation of pancreatic beta functioning. It has been shown that neonatal
treatment with MSG also causes insulin resistance in adult rats (39). In results not shown, a
decrease occurred in tissue insulin sensitivity in 60-day-old MSG-treated rats and in 90-
day-old untreated rats when compared to the youngest ones. However, other obese rodents
from different origins presented noted fasting hyperglycemia when they turn to adult life
(40, 41). Taken together, our data support, at least in part, that beta cells functions
undertake changes to adjust blood glucose levels when peripheral tissues begin to partially
lose their insulin sensitivity. MSG treatment accelerates these changes, without disrupting
glycemic control. As expected, pancreatic islets isolated from MSG-treated and untreated
rats secrete insulin as a dependent manner when stimulated by glucose. However, only
islets from young MSG-obese rats present maximum glucose response lower than lean
ones. Unexpectedly, using the half-maximum response as glucose sensitivity in insulin
secretion process, whereas only high sensitivity in islets of 60-day-old MSG-obese rats was
observed, islets of weaned and adult-obese rats presented similar glucose sensitivity.
Results also demonstrate that glucose sensitivity declines during development, regardless of
obesity onset. Islet functionadjustments, revealed by current results, support the hypothesis
that obesity induced by MSG neonatal treatment does not disrupt insulin secretion control
of beta cells; however changes on glucose-induced insulin secretion by itself does not
explain fasting hyperinsulinemia observed in MSG-obese rats at all ages under analysis. In
MSG and other experimental obese animals, fasting hyperinsulinemia has been attributed to
high vagal activity, while low activity of sympathetic activity has also been observed (42-
44). Vagotomy and atropinization may reduce fasting blood insulin levels in animal and
human obesity (9, 42, 45, 46). Our laboratory also showed that early vagotomy was able to
normalize insulinemia and reduce MSG-obesity onset in rats. In this study it has also been
shown that the cholinergic agonist, carbachol, displayed a low insulinotropic effect on islets
isolated from obese rats; while vagotomy improved the islet cholinergic secretory response
(9). In results not shown in current research, exogenous acetylcholine was not able to raise
blood insulin concentration during intravenous glucose tolerance test in adult MSG-obese
rats. In MSG-obese rats constant high parasympathetic activity released high Ach on beta
cell plasma membrane, which may change number, affinity and/or transduction of
muscarinic signal. In these conditions cholinergic insulinotropic effect may decrease. We
found weak insulin secretion stimulated by Ach in islets isolated from MSG-treated rats.
Maximum response to Ach was progressively decreased during the MSG-obesity onset,
while in lean rats Ach maximum response was preserved. Desensitization to cholinergic
stimulation was observed in islets from lean rats cultivated in the presence of carbachol
(47). Recently, it has been observed in insulin producing clonal line cells that Ach inhibited
glucose-induced insulin secretion throughout activation of muscarinic receptor M2 and M4
subfamilies (48). Mice with knockout of muscarinic receptor M3 are hypoinsulinemic and
their pancreatic islets did not respond to cholinergic stimulation (49-51). Unexpectedly,
regardless to MSG treatment or development effect, pancreatic islets did not change
significantly Ach sensitivity. While Ach induced low insulin secretion, beta cell maintained
constant the sensitivity to neurotransmitter, which might induce insulin oversecretion and
consequent hyperinsulinemia, which is also sustained by a combination of glucose
stimulation and low sympathetic activity, among other factors. In other experimental obese
animals, such as ob/ob mice and Zucker rats, young and adult animals display a high
cholinergic insulinotropic effect (52-55). Recently our laboratory show that, using
muscarinic receptor M2 antagonist, methoctramine, Ach highly potentiate glucose-induced
insulin secretion on islets from adult MSG-obese rats (56). These results suggest that
during the MSG-obesity onset the composition and/or function of muscarinic receptor
subfamilies should be modified on beta cell plasma membrane. Furthermore, weak
insulinotropic Ach effect observed in islets from MSG-obese rats, even in weaned ones,
should be adaptations in the functioning of beta cells to protect organism against
hyperglycemia onset. Development of weaned rats induces changes on metabolism, which
leads to increase fat tissue accretion and decrease in tissue insulin action. These changes do
not disturb the glucose-induced insulin secretion on pancreatic beta, which allow young and
adult rats to sustain normal basal blood glucose levels. Neonatal treatment with MSG early
promoted obesity, enhancing changes observed in untreated rats; while, normoglycemia
was still maintained. Response and sensitivity to acetylcholine are preserved on beta cells
during development of lean rats; while, in MSG-obese animals acetylcholine induces weak
insulinotropic response. However, cholinergic agent sensitivity is also preserved as in beta
cells from obese rats. Findings suggest that beta cells from MSG-obese rats progressively
change their insulin secretion regulation with development. Even with hyperinsulinemia,
obese rats maintain normoglycemia. Studies revealing the mechanisms that protect beta
cells of MSG-obese animals against failure should be very useful towards the prevention
and treatment of human obesity and diabetes.
Acknowledgments
We gratefully acknowledge financial support from CNPq (Brazilian Council for Scientific
and Technological Development).
Figure 1. Effect of early post-weaning development and neonatal MSG treatment on
body fatness, fasting blood glucose and insulin concentration
Values are mean±SEM of 20 animals for both group and ages. Periepididymal fat pad is
presented in Figure 1A. Plasma glucose (1B) and insulin (1C) concentration were obtained
after overnight fasting. *p<0.05 evaluated by Student's t test between control and MSG at
same age. Letters under bars present differences (p<0.05) ANOVA inside of group: a – 30
days; b – 60 days and c – 90 days.
l/is
/m
g
(n 3
10-2.75 10-2.50 10-2.25 10-2.00 10-1.75 10-1.50 10-1.25
10-2.75 10 -2.50 10 -2.25 10-2.00 10 -1.75 10-1.50 10-1.25
10-2.75 10-2.50 10 -2.25 10-2.00 10-1.75 10 -1.50 10-1.25
Glucose [Log M]
Figure 2. Dose – response curve to glucose
Groups of 4 islets were incubated in 1 mL of Krebs solution in different glucose
concentrations expressed as log of concentration in M. Each point represents mean±SEM
from 25 observations. Panels show insulin secretion from islets of 30- (A), 60- (B) and 90-
day-old (C) rat.
re
c
e
S 200
lin
u
s 100
In
%
Acetylcholine [Log M]
Acetylcholine [Log M]
Acetylcholine [Log M]
Figure 3. Dose – response curve to Ach.
Groups of 4 islets were incubated in 1mL of Krebs solution with glucose 8.3mM plus
different acetylcholine concentrations, expressed as log of concentration in M. Insulin
secretion is presented as a percent calculated by secretory response to glucose 8.3 mM
alone, namely 0.45±0.05, 0.69±0.05 and 0.62±0.04 ng/islet, respectively for 30-, 60- and
90-day-old control rats, and 0.36±0.04, 0.75±0.04 and 1.22±0.10 ng/islet to MSG group.
Each symbol represents mean±SEM of 25 observations. Panels show insulin secretion
from islets of 30- (A), 60- (B) and 90-day-old (C) rats.
Half-Maximum Response (EC50)
Maximum Response
Glucose (mM)
Insulin (ng/mL)
17.87 ± 0.89c
15.62 ± 0.83b
5.35 ± 0.50c
4.38 ± 0.38*b
9.92 ± 0.59*ac
2.71 ± 0.11*ac
13.84 ± 0.66a
13.59 ± 1.03b
3.45 ± 0.16a
5.22 ± 0.48*b
Table I. Effect of age development and MSG treatment on glucose concentration that
induces half-maximum insulin secretion and maximum response on isolated islets.
Data are obtained from the dose-response curves shown in Figure 2 and calculated as
indicated in material and methods. * p<0.05 when compared MSG-treated to untreated rats
of same age. Letters represent significant differences with p<0.05 between islets from MSG
or control a, 30-; b, 60- and c, 60-day-old rats.
Half-Maximum Response (EC50)
Maximum Response
Acetylcholine (µM)
Insulin Secretion
(% from glucose 8.3mM)
134.20 ± 11.01*bc
35.29 ± 4.88*a
37.28 ± 3.79*a
Table II. Effect of age development and MSG treatment on Ach concentration that
induces half-maximum insulin secretion and maximum response on isolated islets.
Data are obtained from the dose-response curves shown in Figure 3 and calculated as
indicated in material and methods. * p<0.05 when MSG-treated to untreated rats of same
age are compared. Letters represent significant differences with p<0.05 between islets from
MSG or control a, 30-; b, 60- and c, 60-day-old rats.
Jeanrenaud B: Energy fuel and hormonal profile in experimental obesities. Experientia Suppl 44: 57-76, 1983.
Bray GA: Medical consequences of obesity. J Clin Endocrinol Metab 89: 2583-9, 2004.
Ashcroft FM, Harrison DE, Ashcroft SJ: Glucose induces closure of single potassium channels in isolated rat pancreatic beta-cells. Nature 312: 446-8, 1984.
Malaisse WJ: Glucose-sensing by the pancreatic B-cell: the mitochondrial part. Int J Biochem 24: 693-701, 1992.
Henquin JC: Triggering and amplifying pathways of regulation of insulin secretion by glucose. Diabetes 49: 1751-60, 2000.
Rohner-Jeanrenaud F, Hochstrasser AC, Jeanrenaud B: Hyperinsulinemia of preobese and obese fa/fa rats is partly vagus nerve mediated. Am J Physiol 244: E317-22, 1983.
Fox EA, Powley TL: Regeneration may mediate the sparing of VMH obesity observed with prior vagotomy. Am J Physiol 247: R308-17, 1984.
Bray GA: Hypothalamic and genetic obesity: an appraisal of the autonomic hypothesis and the endocrine hypothesis. Int J Obes 8 Suppl 1: 119-37, 1984.
Balbo SL, Bonfleur ML, Carneiro EM, Amaral ME, Filiputti E, Mathias PC: Parasympathetic activity changes insulin response to glucose and neurotransmitters. Diabetes Metab 28: 3S13-7; discussion 3S108-12, 2002.
Gilon P, Henquin JC: Mechanisms and physiological significance of the cholinergic control of pancreatic beta-cell function. Endocr Rev 22: 565-604, 2001.
Mathias PC, Carpinelli AR, Billaudel B, Garcia-Morales P, Valverde I, Malaisse WJ: Cholinergic stimulation of ion fluxes in pancreatic islets. Biochem Pharmacol 34: 3451-7, 1985.
Boschero AC, Szpak-Glasman M, Carneiro EM, et al.: Oxotremorine-m potentiation of glucose-induced insulin release from rat islets involves M3 muscarinic receptors. Am J Physiol 268: E336-42, 1995.
Saxton AM, Eisen EJ: Genetic analysis of brown adipose tissue, obesity and growth in mice. Genetics 106: 705-18, 1984.
Heywood WE, Mian N, Milla PJ, Lindley KJ: Programming of defective rat pancreatic beta-cell function in offspring from mothers fed a low-protein diet during gestation and the suckling periods. Clin Sci (Lond) 107: 37-45, 2004.
Waterland RA, Garza C: Early postnatal nutrition determines adult pancreatic glucose-responsive insulin secretion and islet gene expression in rats. J Nutr 132: 357-64, 2002.
Martins PA, Sawaya AL: Evidence for impaired insulin production and higher sensitivity in stunted children living in slums. Br J Nutr 95: 996-1001, 2006.
Bliss CR, Sharp GW: Glucose-induced insulin release in islets of young rats: time-dependent potentiation and effects of 2-bromostearate. Am J Physiol 263: E890-6, 1992.
Grill V, Asplund K, Hellerstrom C, Cerasi E: Decreased cyclic AMP and insulin response to glucose in isolated islets of neonatal rats. Diabetes 24: 746-52, 1975.
Reaven EP, Gold G, Reaven GM: Effect of age on glucose-stimulated insulin release by the beta-cell of the rat. J Clin Invest 64: 591-9, 1979.
Reaven GM, Reaven PD: Effect of age on glucose oxidation by isolated rat islets. Diabetologia 18: 69-71, 1980.
Launer LJ: Diabetes and brain aging: epidemiologic evidence. Curr Diab Rep 5: 59-63, 2005.
Malaisse WJ, Malaisse-Lagae F, Coleman DL: Insulin secretion in experimental obesity. Metabolism 17: 802-7, 1968.
Beck P, Koumans JH, Winterling CA, Stein MF, Daughaday WH, Kipnis DM: Studies Of Insulin And Growth Hormone Secretion In Human Obesity. J Lab Clin Med 64: 654-67, 1964.
Barzilai N, Rossetti L: Relationship between changes in body composition and insulin responsiveness in models of the aging rat. Am J Physiol 269: E591-7, 1995.
Herzog CD, Nowak KA, Sarter M, Bruno JP: Microdialysis without acetylcholinesterase inhibition reveals an age-related attenuation in stimulated cortical acetylcholine release. Neurobiol Aging 24: 861-3, 2003.
Phillips RJ, Kieffer EJ, Powley TL: Aging of the myenteric plexus: neuronal loss is specific to cholinergic neurons. Auton Neurosci 106: 69-83, 2003.
Persson-Sjogren S, Forsgren S, Lindstrom P: Vasoactive intestinal polypeptide and pituitary adenylate cyclase activating polypeptide: Effects on insulin release in isolated mouse islets in relation to metabolic status and age. Neuropeptides: 2006.
Hirata AE, Alvarez-Rojas F, Carvalheira JB, Carvalho CR, Dolnikoff MS, Abdalla Saad MJ: Modulation of IR/PTP1B interaction and downstream signaling in insulin sensitive tissues of MSG-rats. Life Sci 73: 1369-81, 2003.
Moreno G, Perello M, Camihort G, et al.: Impact of transient correction of increased adrenocortical activity in hypothalamo-damaged, hyperadipose female rats. Int J Obes (Lond) 30: 73-82, 2006.
Trinder P: Determination of blood glucose using an oxidase-peroxidase system with a non-carcinogenic chromogen. J Clin Pathol 22: 158-61, 1969.
Scott AM, Atwater I, Rojas E: A method for the simultaneous measurement of insulin release and B cell membrane potential in single mouse islets of Langerhans. Diabetologia 21: 470-5, 1981.
Gravena C, Mathias PC, Ashcroft SJ: Acute effects of fatty acids on insulin secretion from rat and human islets of Langerhans. J Endocrinol 173: 73-80, 2002.
Kazdova L, Fabry P: Fat cells in ontogenesis. Adv Exp Med Biol 53: 247-56, 1975.
Holm G, Jacobsson B, Bjorntorp P, Smith U: Effects of age and cell size on rat adipose tissue metabolism. J Lipid Res 16: 461-4, 1975.
Holliday MA, Potter D, Jarrah A, Bearg S: The relation of metabolic rate to body weight and organ size. Pediatr Res 1: 185-95, 1967.
Wolters GH, Wiegman JB, Konijnendijk W: Effect of glucose stimulation on 45calcium uptake and total calcium content of pancreatic islets of fed and fasted rats and obese hyperglycemic mice. Diabetes 32: 124-9, 1983.
Rhodes CJ: Type 2 diabetes-a matter of beta-cell life and death? Science 307: 380-4, 2005.
Macho L, Fickova M, Jezova, Zorad S: Late effects of postnatal administration of monosodium glutamate on insulin action in adult rats. Physiol Res 49 Suppl 1: S79-85, 2000.
Thirone AC, Carvalheira JB, Hirata AE, Velloso LA, Saad MJ: Regulation of Cbl-associated protein/Cbl pathway in muscle and adipose tissues of two animal models of insulin resistance. Endocrinology 145: 281-93, 2004.
Furnsinn C, Komjati M, Madsen OD, Schneider B, Waldhausl W: Lifelong sequential changes in glucose tolerance and insulin secretion in genetically obese Zucker rats (fa/fa) fed a diabetogenic diet. Endocrinology 128: 1093-9, 1991.
Lavine RL, Voyles N, Perrino PV, Recant L: Functional abnormalities of islets of Langerhans of obese hyperglycemic mouse. Am J Physiol 233: E86-90, 1977.
Inoue S, Bray GA: The effects of subdiaphragmatic vagotomy in rats with ventromedial hypothalamic obesity. Endocrinology 100: 108-14, 1977.
Scheurink AJ, Balkan B, Strubbe JH, van Dijk G, Steffens AB: Overfeeding, autonomic regulation and metabolic consequences. Cardiovasc Drugs Ther 10 Suppl 1: 263-73, 1996.
Valensi P, Doare L, Perret G, Germack R, Paries J, Mesangeau D: Cardiovascular vagosympathetic activity in rats with ventromedial hypothalamic obesity. Obes Res 11: 54-64, 2003.
Teff KL, Townsend RR: Early phase insulin infusion and muscarinic blockade in obese and lean subjects. Am J Physiol 277: R198-208, 1999.
Ahren B, Lundquist I: Modulation of basal insulin secretion in the obese, hyperglycemic mouse. Metabolism 31: 172-9, 1982.
Yamazaki H, Philbrick W, Zawalich KC, Zawalich WS: Acute and chronic effects of glucose and carbachol on insulin secretion and phospholipase C activation: studies with diazoxide and atropine. Am J Physiol Endocrinol Metab 290: E26-E33, 2006.
Miguel JC, Abdel-Wahab YH, Mathias PC, Flatt PR: Muscarinic receptor subtypes mediate stimulatory and paradoxical inhibitory effects on an insulin-secreting beta cell line. Biochim Biophys Acta 1569: 45-50, 2002.
Zawalich WS, Zawalich KC, Tesz GJ, et al.: Effects of muscarinic receptor type 3 knockout on mouse islet secretory responses. Biochem Biophys Res Commun 315: 872-6, 2004.
Yamada M, Miyakawa T, Duttaroy A, et al.: Mice lacking the M3 muscarinic acetylcholine receptor are hypophagic and lean. Nature 410: 207-12, 2001.
Duttaroy A, Zimliki CL, Gautam D, Cui Y, Mears D, Wess J: Muscarinic stimulation of pancreatic insulin and glucagon release is abolished in m3 muscarinic acetylcholine receptor-deficient mice. Diabetes 53: 1714-20, 2004.
Atef N, Brule C, Bihoreau MT, Ktorza A, Picon L, Penicaud L: Enhanced insulin secretory response to acetylcholine by perifused pancreas of 5-day-old preobese Zucker rats. Endocrinology 129: 2219-24, 1991.
Persson-Sjogren S, Elmi A, Lindstrom P: Effects of leptin, acetylcholine and vasoactive intestinal polypeptide on insulin secretion in isolated ob/ob mouse pancreatic islets. Acta Diabetol 41: 104-12, 2004.
Persson-Sjogren S, Lindstrom P: Effects of cholinergic m-receptor agonists on insulin release in islets from obese and lean mice of different ages: the importance of bicarbonate. Pancreas 29: e90-9, 2004.
Chen NG, Romsos DR: Persistently enhanced sensitivity of pancreatic islets from ob/ob mice to PKC-stimulated insulin secretion. Am J Physiol 272: E304-11, 1997.
Grassiolli S, Gravena C, Mathias PC: Muscarinic M2 receptor is active on pancreatic islets from hypothalamic obese rat. Eur J Pharmacol in press: 2006.
Transplantation of Pancreatic Islets From Hypothalamic Obese Rats
Corrects Hyperglycemia of Diabetic Rats
P.C. de Freitas Mathias, S. Grassiolli, D.N. Rocha, D.X. Scomparin, and C. Gravena
Pancreatic islets isolated from adult obese rats, obtained by neonatal treatment withmonosodium L-glutamate (MSG), oversecrete insulin stimulated by glucose concentra-tion. Whereas adult MSG obese rats are hyperinsulinemic, their pancreatic islets stillsecrete insulin after high glucose demand. This is crucial so that the animals do not becomehyperglycemic. Islets from MSG obese rats were implanted in diabetic donor rats so thatthe capacity of islets in regulating blood glucose concentration could be evaluated.
Hyperglycemic (glucose 22 to 34 mmol/L) rats obtained with streptozotocin (STZ)treatment were used as recipients. Islet donors consisted of control adult and MSG obeserats. Only 600 islets were transplanted via the portal vein to diabetic rats. During 4 daysafter the transplant, fed blood glucose was monitored. After 12 hours of fasting the ratswere killed; their blood samples were used to measure glucose and insulin concentration;retroperitoneal fat pads were isolated and weighed to estimate body fat. Transplantedislets from MSG obese rats decreased of fed glucose levels by 34% in diabetic rats (P ⬍.05); however, glucose levels still remained twofold higher than those of intact controls(P ⬍ .05). Similar to MSG islets, islets grafts from control rats provoked the same effectsin diabetic rats. High fasting blood glucose and low insulin levels of diabetic rats werecorrected by islet grafts. Transplantations were able to recover 40% of fat in diabetic rats.
The results demonstrated that islets from MSG obese rats may regulate blood glucoseconcentrations in diabetic rats, and suggesting that their function was not permanentlyaltered by the onset of obesity.
HYPERINSULINEMIA and insulin resistance are Streptozotocin-Induced Diabetes
hallmarks of obesity with high risks of developing
In control rats diabetes was induced by streptozotocin (STZ; 80
diabetes. Unlike other animal models of obesity, neonatal
mg/kg intraperitoneally). Blood glucose concentrations of 22 to 34
treatment with monosodium L-glutamate (MSG) induced
mmol/L were used to select diabetic rats at 4 days after STZ
hypothalamic obesity in rats, which did not cause the onset
injections, these animals were used as recipients.
of hyperglycemia. Upon glucose stimulation, pancreaticislets from adult MSG rats oversecrete It has been
Islets Isolation and Transplantation
suggested that changes in glucose-induced insulin secretionamong MSG obese rats may be permanent adjustments in
Islets (600) isolated by the collagenase technique and purified by
the function of beta To test this hypothesis, islets of
centrifugation were handpicked and injected into the main branch
MSG obese rats were transplanted into diabetic rats toassess the capacity of grafts to control blood glucose levels.
From the State University of Maringá, Department of Cell
Biology and Genetics, Marangá, Brazil.
Supported by the Brazilian Council for Scientific and Techno-
MATERIALS AND METHODS
logical Development (CNPq) and the Paraná Araucária Founda-
Neonatal male Wistar rats received subcutaneous injections of
Address reprint requests to Paulo Cezar de Freitas Mathias,
MSG (4 g/kg) during the first 5 days of life. Control rats received
PhD, State University of Maringá, Department of Cell Biology
equimolar amounts of saline solution. Donors used in the protocols
and Genetics, Av. Colombo 5790, Bloco H-67, Sala 019, Ma-
were 80- to 90-day-old rats.
rangá, Paraná 87020-900, Brazil. E-mail: [email protected]
2007 by Elsevier Inc. All rights reserved.
0041-1345/07/$–see front matter
360 Park Avenue South, New York, NY 10010-1710
Transplantation Proceedings, 39, 193–195 (2007)
DE FREITAS MATHIAS, GRASSIOLLI, ROCHA ET AL
Table 1. Effect of Islet Transplantation on Fasting Blood Glucose, Insulin, and Retroperitoneal Fat Accumulation
Fat (g/100 g body weight) Retroperitoneal
0.80 ⫾ 0.05bcde
1.40 ⫾ 0.08acde
27.60 ⫾ 3.15abde
0.12 ⫾ 0.03abde
Data represent mean ⫾ SEM of 10 to 16 rats for each experimental animal group. Letters represent significant differences (P ⬍ .05) between rat groups: a, intact
control; b, intact MSG; c, diabetic (STZ); d, diabetic transplanted with islets from control (STZ ⫹ Control); and e, diabetic transplanted with islets from MSG (STZ ⫹MSG). Blood insulin levels were not detectable (ND) to radioimmunoassay in diabetic rats.
of the portal vein under ketamine and xylazine anesthesia (55 and
.05). STZ treatment produced a fourfold increase in plasma
8 mg/Kg, respectively).
glucose concentration and a sevenfold decrease in fataccumulation in the retroperitoneal pads, compared with
the controls (P ⬍ .05; Table 1). Islet grafts decreased fasting
During the next 4 days after transplantation, blood samples were
glucose and insulin levels to the same values as those in
collected from the tail tip of fed animals to measure plasma glucose
control rats, even though the islets originated from donor
levels. After 12-hour fasting, all rats underwent anesthesia for
animals, from controls, or from MSG treated animals.
sacrifice by cervical dislocation. Isolated retroperitoneal fat pads
Table 1 also shows that the two islet grafts recovered the
were washed and weighed to estimate body fat. Blood samples were
retroperitoneal fat content by 40% compared with that in
used to measure plasma glucose and insulin concentrations.
control rats. Analyzing fed plasma glucose levels, shows that 1 day after surgery, the grafted islets from
Statistical Analysis
controls or from MSG obese animals, decreased hypergly-
Results are shown as mean values ⫾ standard error of the mean
cemia in diabetic rats by 34% (P ⬍ .05). However, grafts
(SEM). Analysis of variance tested differences for more than two
were not effective to reach the levels of control rats, which
different groups. In the case of analyses with a significant F, the
were over 20% greater (P ⬍ .05). Figure 1 shows that after
differences between mean values were evaluated by a Bonferroni
surgery, including sham-operated controls, there was a 15%
t test. P values less than .05 were considered statistically significant.
decrease in glucose levels, (P ⬍ .05).
Tests were performed using GraphPad Prism version 3.02 forWindows (GraphPad Software).
Since neonatal treatment with MSG produced obesity in
adult rats with fasting hyperinsulinemia but not hypergly-
shows that neonatal MSG treatment caused a 75%
cemia, we confirmed the previous Pancreatic islets
increase in fat accumulation in the retroperitoneal pad
isolated from MSG obese rats oversecrete insulin upon
compared with that in control rats (P ⬍ .05). MSG treat-
stimulation by glucose, which, at least in part, supports the
ment induced increased fasting plasma insulin (twofold)
observation of Islet grafts from obese or
compared with the insulin levels of untreated animals (P ⬍
from lean rats maintained fasting normoglycemia but were
Islet graft effect on fed
blood glucose concentration.
Data represent mean ⫾ SEM of10 to 16 rats for each experi-mental animal group. Symbolsrepresent significant differences(P ⬍ .05) for *diabetic (STZ) ⫻control; ⌿diabetic transplantedwith islets from control (STZ ⫹control) or diabetic transplantedwith islets from MSG (STZ ⫹MSG) ⫻ control; and ⍀diabetictransplanted with islets fromcontrol (STZ ⫹ control) or dia-betic transplanted with isletsfrom MSG (STZ ⫹ MSG) ⫻ Di-abetic (STZ).
PANCREATIC ISLET TRANSPLANTATION
ineffective to decrease fed blood glucose. Fasting blood
mental protocol for islet transplantation, including only 4
glucose is related to tissue insulin sensitivity and fasting
days of monitoring, was developed to avoid the interference
blood insulin levels, which were maintained by grafts to
of neural control of insulin secretion. Blood glucose fluctu-
levels like those of control animals. Although the results
ations are the main signal to control insulin secretion in
indicated that transplanted islets secreted insulin upon
transplanted diabetic rats. It has been observed that vagal
stimulation with a low glucose concentration as found in the
activity has a strong impact on blood insulin levels of obese
fasting state, they failed to meet the demand imposed by
and lean rats.The present results suggest that islets
high glucose concentrations in a postprandial situation. It
obtained from obese rats that underwent neonatal treat-
was remarkable that the low insulin secretion from grafts
ment with MSG, even in small numbers, produced similar
induced a significant regain of fat accretion. Results not
fasting blood glucose levels as controls. However, the islets
shown in this article here suggested that as expected rats
did not maintain their capacity to oversecrete insulin when
transplanted with islets from control or MSG obese rats
freshly isolated from obese animals. The mechanisms by
showed higher tissue insulin sensitivity than those from
which islets lose their oversecretory function remain to be
intact control rats. Since the insulin sensitivity of white fat
tissue is preserved in MSG obese rats, one may considerwhy the beta cells of obese rats are not damaged and
maintain normoglycemia.The number of islets implanted
1. Marçal AC, et al: The dual effect of isoproterenol on insulin
into diabetic rats was lower than that needed to better
release is supressed in pancreatic islets from hypothalamic obese
regulate blood glucose levels, namely, more than 1000 to
rats. Endocrine 29:in press, 2006
2. Hirata AE, et al: Modulation of 1R/PTP1B interaction and
However, the low islet mass was used to observe
downstream signaling in insulin sensitive tissues of MSG-rats. Life
whether the oversecretory potential of islets from MSG
Sci 73:1369, 2003
obese rats might also control fed blood glucose. In addition,
3. Balbo SL, et al: Parasympathetic activity changes insulin
it has been observed that part of the implanted islets failed
response to glucose and neurotransmitters. Diabetes Metab 28:
to secrete insulin or were destroyed within a few days of the
3S13, 2002 (Discussion 3S108)
4. Morsiani E, et al: Repeated intraportal injections of subthera-
operation,which may indicate a low capacity of the graft to
peutic islet cell isografts restore normoglycemia in streptozotocin-
control fed blood glucose concentrations. While the same
diabetic rats. Cell Transplant 6:17, 1997
results were obtained with grafts from donor control rats, it
5. Fritschy WM, et al: The efficacy of intraperitoneal pancreatic
may be argued whether islets from MSG obese rats are
islet isografts in the reversal of diabetes in rats. Transplantation52:777, 1991
more fragile than control islets. It has been reported that 5
6. Adeghate E: Pancreatic tissue grafts are reinnervated by
days after grafting, islets recover neural terminals, which
neuro-peptidergic and cholinergic nerves within five days of trans-
modulate glucose-induced insulin secretion.The experi-
plantation. Transpl Immunol 10:73, 2002
Glucose-induced insulin secretion changes as from weaning through adult
life in normal and MSG-obese rats
Sabrina Grassiolli, Dionizia Xavier Scomparin, Ana Eliza Andreazzi, Sandra
Lucinei Balbo, Clarice Gravena and Paulo Cezar de Freitas Mathias
Laboratory of Secretion Cell Biology, Department of Cell Biology and Genetics,
State University of Maringá, 87020-900 Maringá PR Brazil
Corresponding Author
Paulo Cezar de Freitas Mathias
Laboratory of Secretion Cell Biology
Department of Cell Biology and Genetics
State University of Maringá
Avenida Colombo 5790
87020-900 Maringá PR BRAZIL
Phone/fax: 00 – 55- 44 - 3261 - 4892
E-mail: [email protected]
Running title: Insulin secretion in early post-weaned MSG-rats
Key words: MSG obesity, insulin secretion, early post-weaned development,
pancreatic islets, insulin sensitivity
Abstract
Background: Pancreatic islets from obese adult rats oversecrete insulin. The aim
of present work is to know whether perinatal development has impact over beta
cell function and its relation to MSG obesity. Methods: Obese rats were obtained
by MSG neonatal treatment. Treated and untreated rats were divided by age:
weaned (30), young (60) and adult (90-day-old). Animals were submitted to
intravenous glucose tolerance test. Glucose-induced insulin secretion (GIIS) on
isolated islets was studied. Results: While progressive fat accumulation was
reported in the two groups, MSG-rats showed a higher increase. Whereas fasting
glucose failed to change in both groups, insulin levels became higher. Glucose
tolerance was not different in lean and obese rat development, whereas obesity
induced glucose intolerance. Adult rats showed decreased insulin sensitivity;
however, it was pronounced in obese ones. Islets showed a dose-dependent curve
to GIIS, while responses were higher in obese ones. At low glucose
concentrations, islets from younger rats secrete more insulin than adult ones;
however, islets from aged rats showed increased response to high glucose
concentrations. Conclusions: While, lean and obese rats present progressive
increased fatness and decreased insulin sensitivity during development, unlike
other obesity models, MSG-rats does not became diabetic.
Blood insulin levels change during aging and their control is consonant to
changes in the metabolism. These adapting mechanisms affect the glucose-
induced insulin secretion process from the pancreas.
As glucose, other different nutrients, such as aminoacids, fat acids and
their metabolites, stimulate also insulin secretion on pancreatic beta cells. These
secretagogues induce an increase in cell metabolism and subsequent ATP
production. Potassium ATP sensitive channels (KATP) are inactivated by an
increase in ATP/ADP ratio. Now this triggers membrane depolarization and
subsequently the activation of dependent Ca2+ channels-voltage that increases
intracellular calcium concentration. High cytosolic free calcium is an intracellular
signal that triggers insulin secretion events. This is accepted as a triggering
pathway that permits insulin to be secreted into the blood stream. However,
experimental evidences indicate that glucose may also be stimulating insulin
secretion by alternative pathways to KATP channels [1].
Reduced insulin secretion has been reported in pancreatic islets from
neonatal, post-weaning and young rats as a response to high glucose stimulation,
when compared to islets of adult rats [2,3]. A decline in insulin secretory response
from pancreatic islets with aging has been observed [4-6]. Aging is a risk factor
in the development of degenerative diseases with high prevalence worldwide,
such as obesity and non-insulin-dependent diabetes (NIDDM). It has been
demonstrated that in 60-year-old people or over the prevalence of NIDDM
increases when compared to that in younger people [7]. Among the hallmarks of
diabetes and obesity, fasting hyperinsulinemia, hyperglycemia and insulin
resistance have been encountered. Glucose-induced insulin secretion is also
impaired in obese and diabetic patients, both young and old. Since diabetes
results from an imbalance between insulin sensitivity and beta cell function, either
or both may worsen with age. Since the 1960s obesity is associated with increased
insulin secretion [8,9], it is evident that insulin oversecretion in obesity is not
caused by the obesity per se but by concomitant reduced insulin sensitivity. The
mechanism underling the islet adaptation to insulin resistance, or rather, how beta
cell may experience a reduction in insulin sensitivity, is not yet known. Insulin
resistance increases in aging rats [10]. However, whether alterations in insulin
sensitivity and pancreatic beta cell function exist and progress in the early stage of
development, from the post-weaning phase through adult life, are still unknown.
Perinatal life is important to the development of the whole organism and of the
functioning of the pancreatic islets. Any nutritional disturbances or other injuries
during fetus or neonatal life, in human and in laboratory animals, may cause
permanent metabolic alterations, which lead to obesity and diabetes, among other
chronic diseases, in adult life [6,11,12]. Administrations of monosodium L-
glutamate (MSG) to rodents' suckling pups promote the death of neurons in
hypothalamic areas and induce changes in the development of the central nervous
system (CNS). Adult MSG-treated rats and mice exhibit disturbances in body
weight which lead to increased adiposity, hyperinsulinemia and insulin resistance.
Unlike other obese rodents, such as those from genetic origin or from electrolytic
lesions in CNS, overeating in MSG-rodents does not occur. In spite of
hyperinsulinemia and insulin resistance, adult MSG-rats are normoglycemic [13-
15]. It is surprising that adult MSG-obese rats do not develop fasting
hyperglycemia and diabetes. While pancreatic islets from these obese rats
oversecreted insulin stimulated by high glucose concentration [14], their beta cells
maintained the production, storage and insulin exocytosis to avoid the onset of
The aim of current research is to investigate: i) the glucose-stimulated
insulin secretion from pancreatic islets and insulin resistance during the
development of rats, from the post-weaning phase through adult life, and ii)
whether onset of MSG-obesity speeds up damage to the functioning of beta cells
and to insulin peripheral action.
Materials and Methods
Animals and obesity: Neonate Wistar rats received monosodium-L-glutamate
(MSG) injections intradermically during the first five days of life, with a dose
consisting of 4g/Kg body weight (BW). Control animals received equimolar
saline solution. The two groups of animals were weaned when 21 days old. All
animals were housed under control conditions with 12 hours light-dark luminosity
cycle (07:00 to 19:00 h), at 21±2ºC. Water and standard rodent chow (Nuvital-
Curitiba-Brazil) were supplied ad libitum. Only males were used in the
experimental protocols. MSG-treated and control rats were divided into three
groups: post-weaned (30 days), young (60 days) and adult rats (90 days), for all
protocols. Rats from all groups were anesthetized with ketamine and xylasine (55
and 8 mg/Kg BW, respectively) and sacrificed by cervical dislocation.
Periepididymal fat pads were removed, washed and weighed to estimate obesity
induced by MSG treatment. Lee index [body weight (g)1/3/nasal-anal length (cm) x
1000], employed as a predictor of obesity in MSG-rodents, was calculated [16].
Glucose Tolerance Test: Under anesthesia, a silicone cannula was implanted into
the remaining rats' left jugular vein and attached to the animals' back.
Heparinized saline solution (50 IU heparin in saline 0.9%/ml) was previously
injected into the cannula to avoid blood clotting. Intravenous glucose tolerance
tests (ivGTT) were performed at 8:00 after a 12h fast (19:00-07:00); rats were
injected with a glucose load (1g /Kg BW) through the cannula, without any
anesthesia. Blood samples (300 µl) were collected from the same cannula
sequentially before glucose load (t0) and 5 (t5), 15 (t15), 30 (t30) and 60 (t60)
min after glucose injection [19]. Samples were then centrifuged; the plasma was
separated and stored at 20ºC for posterior dosage of glucose concentration by the
glucose oxidase method (Kit-Bio Diagnostic Chemistry Industry®) and insulin by
RIA. Insulinogenic index (∆ insulinemia/∆ glycemia) was calculated by the area
under the glycemia and insulinemia curves (AUC), during 60min of ivGTT, with
zero time as base line.
Pancreatic Islets Isolation: Isolation of islets from the rats' pancreas was
performed as previously described [17], with adaptations. Rats from all groups
were anesthetized and had their abdominal wall cut and opened. A 10 ml Hank's
buffered saline solution (HBSS) containing collagenase type V (0.7 mg/ml, Sigma
Chemical CO., St. Louis, MO) was injected into the common bile duct of the rats.
The pancreas, swollen with the collagenase solution, was quickly excised and
incubated in a plastic culture bottle for 15 min, at 37ºC. The suspension was then
filtered with a 0.5mm metal mesh and washed with HBSS, including 0.12%
bovine serum albumin fraction V (BSA) in 5 continuous washings. Islets were
collected with the aid of a microscope. At least 3 rats were used to obtain a
pancreatic rat pool for each animal group.
Glucose-induced insulin secretion in pancreatic islets: Batches of 4 islets were
pre-incubated for 60 min in 1mL of normal Krebs solution containing: 120mM
NaCl; 4.8mM KCl; 2.5mM CaCl2; 1.2mM MgCl2 and 24mM NaHCO3, and 5.6
mM glucose. This solution was gassed with O2/CO2 (95/5%) to maintain pH 7.4
and supplemented with BSA 0.12%. After this period the islets were submitted to
different concentration of glucose: 5.6; 8.3; 11.6; 16.7 and 20.0mM for another 60
min of incubation. Samples of incubation media were taken, frozen and stored
until secreted insulin by radioimmunoassay (RIA) could be measured [18].
The Ethical Committee for Animal Experiments of the State University of
Maringá, Maringá PR Brazil approved the described protocols.
Chemicals
[I125] Human insulin recombinant was acquired from Pharmacia (São Paulo, BR)
and routine reagents were purchased from Sigma, unless otherwise specified.
Statistical Analysis
Results were given as mean ± SEM. Data from control groups and MSG-treated
rats of the same age were submitted to Student's t test. Variance Analysis
(ANOVA) was used to test differences between groups of control and MSG-rats
at different ages. In the case of analyses with a significant F, the differences
between means were evaluated by Bonferroni t-test. P values less than 0.05 were
considered statistically significant. Tests were undertaken using GraphPad Prism
version 3.02 for Windows (GraphPad Software®).
Lee index, as obesity predictor, showed that development causes a 5%
increase after 60 days of birth, whereas after 90 days the same values have been
reported for weaned rats, to both groups, p<0.05, as shown in Figure 1A. While
neonatal MSG treatment induced a 9% rise in Lee index for 3 ages when
compared to respective controls, p<0.05. When fat accumulation in
periepididymal pad is used as body fatness estimator, a progressive increase by
50-100% from weaned to adult rats, whether MSG treated or untreated, p<0.001,
occurs; however MSG treatment heightened the development effect, as Figure 1B
shows. Panel 1C presents fasting glucose plasma concentration, or rather, in
normal and treated MSG rats the glycemia does not change during development,
with the exception of a young MSG-rat which developed a rise in glucose plasma
levels when compared to those of normal young rats. Unlike glycemia, fasting
insulin plasma concentration increases by 60% in adult normal rats when
compared to that in weaned or young rats with similar insulin plasma rates.
However MSG treatment provoked progressive increment of insulin levels during
the development, reaching 7 and 4 fold in adult rats when compared respectively
to weaned and to young ones, p<0.001.
Figure 2 shows plasma glucose (Panels A, B and C) and insulin
increments (Panels D, E and F) which rise progressively after glucose load in
ivGTT during development. Effect has been more evident in MSG-treated
animals. Calculating AUC for glucose and insulin concentration during all ivGTT,
glucose levels increased by 40% from weaned to young normal rats, p<0.05,
whereas normal adult rats have the same values as those of younger animals, as
Table 1 shows. Although glucose AUC in MSG-rats is higher by 50% than that in
controls, development did not affect it, as Table 1 demonstrates. Insulin AUC in
adult normal rats increased 2.6 and 5.2 fold when compared to weaned and young
ones, respectively, p<0.05. Table 1 also shows that the effect of development on
insulin AUC was progressive and more pronounced in MSG-rats. Insulin AUC in
MSG-rats was higher than that in untreated rats, at all ages, reaching 3 fold for
adult animals, p<0.05.
Insulinogenic index increases during development in normal rats, showing
3 fold high in adult rats, when compared to those in young ones. Table 2 also
shows that MSG-rats have a progressive increase in insulinogenic index by 3 fold
during development, when compared according to age, p<0.01. MSG treatment
induced double increase of insulinogenic index when compared to that in
untreated rats, p<0.05, with the exception of weaned rats whose index did not
change, as Table 2 demonstrates.
Figure 3 shows that pancreatic islets isolated from weaned, young and
adult rats, treated or not with MSG, respond to glucose secreting insulin in a dose
dependent manner. A low glucose concentration, 2.8 and 5.6 mM, considered to
be fasting glucose blood levels, stimulates the same insulin secretion response on
islets of normal and MSG-rats. However, islets of MSG-rats showed higher
insulin release than those of controls when stimulated by elevated glucose
concentration, 8.3-16.7 mM. So that the development effect on glucose-induced
insulin secretion from islets of MSG-treated and untreated rats may be better
analyzed Figure 4 is given to show that islets of weaned and young rats secrete
more insulin than adult ones to glucose at 2.8-8.3 mM, whereas islets from adult
rats show a better response than those of two young rat groups when stimulated
by high glucose concentrations, 16.7 and 20.0 mM. These effects occur in spite of
and regardless of MSG treatment.
Discussion
As expected, development of normal rats from the weaning phase through
adulthood induced an increase in fat accumulation. Although most data were
obtained from many different age development stages, mostly came from aging
rats [20], including 6-month-old rats as young [21]. Results confirm previous data
that fat tissue accretion increased during early post-weaned development phase
[22]. MSG treatment provoked pronounced increase in fat accumulation during
development from weaning (30-day-old rats) to adulthood (90-day-old rats). It has
been shown that MSG neonatal treatment of rodents induces obesity at adult age,
after 90 days from birth [13,23-25]. Studies focused on the fatness of young post-
weaned MSG rats are rare. While 30-day-old MSG-rats showed increased
lipogenesis activity, enhanced fat tissue accumulation has not been found [26].
Other studies did not find any fat increase in retroperitoneal and epididymal
tissues in 30- and 40-day-old rats which were treated with MSG in the neonatal
phase [27,28]. Discrepant data are a consequence of MSG doses used: 2 mg/ Kg
BW. In current study 4 mg MSG/Kg BW were used. Instead of relative data, fat
mass/ BW mass, fat accumulation was expressed in absolute values. The type of
results which estimates fatness is very important to MSG-animals. Neonatal
treatment with MSG induces severe reduction of growth hormone levels [29] and
produces shorter and lighter animals than control rats [25]. Most studies with
similar animal model use the Lee index as a predictor of obesity which is actually
well correlated to fat periepididymal pad and to total fat carcass [16]. Lee index
data did not show any effect of development on the two animal groups, while
MSG treatment induced a rise when untreated animals were compared. Results
indicate that Lee index may not be recommended to observe the progressive fat
accumulation during rat development. It is not surprising that early studies had the
same values in Lee index for 21- and 130-day-old rats [30].
In current research, a rise in fasting insulin has been reported during the
development of weaned rats with a simultaneous increase in fatness. It has also
been observed that insulinogenic index increased during development.
Insulinogenic index has been used to estimate insulin sensitivity in peripheral
tissues, or rather, tissues had less insulin sensitivity in proportion to rise in
insulinogenic index [19,31]. Aging is frequently associated with increase in fat
mass and loss of insulin sensitivity, factors which have been observed in humans
and animals [10,32]. Our results indicate that decrease in insulin sensitivity is an
adjustment to progressive tissue fat accumulation, which may be a normal process
during early post-weaned development in sedentary rats. 50-day-old rats have
been marked by extreme changes at sexual hormone levels and metabolism which
lead to puberty [33]. Insulin sensitivity actually decreases in puberty [34].
Neonatal MSG treatment induced high accumulation in weaned, young
and adult rats, coupled to a substantial increase in fasting insulin levels and a
decrease in insulin sensitivity. Results show that during development MSG-rats
presented the same oscillating values as those in untreated rats; however, the high
rates may be attributed to MSG obesity onset. As a rule, hyperinsulinemia and
insulin resistance have been reported only in adult MSG-obese rats [13,15,24,35].
While neonatal MSG treatment has induced changes in fasting insulin, fat
accretion and insulin sensitivity, these conditions are still insufficient to alter
fasting glucose levels. Furthermore, weaned MSG-rats are not insulin resistant
since obesity is already installed. Owing to the fact that insulin is a hormone with
high lipogenic action, an increase in insulin secretion may trigger an increase in
lipid synthesis and insulin resistance observed in all animal models of obesity
Beta cell functioning may be of main importance to adjustment
mechanisms which are involved during development. Pancreatic islets from
weaned and young rats, MSG-treated or untreated, stimulated by low glucose
concentration, release more insulin than islets isolated from adult rats. The
number and mass of rat pancreatic islets are higher during the first month of life
[37]. This fact may be attributed, at least in part, to pancreatic beta cell
proliferation observed in the period [38]. Overall data indicate that oscillations of
insulin levels are associated directly to obesity and insulin resistance observed in
90-day-old adult rats. However, weaned (30-day-old) and young (60-day-old) rats
do not develop these abnormalities and their islets are subjected to low functional
demand to release insulin. On other hand, adult rat islets, treated and untreated
with MSG, release more insulin when stimulated by high glucose concentration,
when compared to younger ones. With regard to insulin resistance, high fat
accumulation and high fasting insulin, the most recent results indicate that during
development functional adjustments occur with high glucose insulinotropic
effects on islets and with the maintenance of glucose homeostasis. To support the
hypothesis that pancreatic islets adjust their functioning according to the
metabolic state, it has been shown that islets from MSG-obese rats with high fat
tissue accumulation and insulin resistance release more insulin in response to high
glucose concentration. It has also been demonstrated that constantly
overstimulated, beta cells fail in glucose-induced insulin secretion [39], similar to
other animal models of obesity, such as ob/ob mice and Zucker rats [40,41].
These obese adult animals are hyperinsulinemic, insulin resistant and
hyperglycemic. Unlike other obesity models, in tough MSG-rats, subjected to the
same impact of development similar to untreated rats, islets surpass the
development and obesity effects and still secrete insulin to preserve
normoglycemia and avoid the onset of diabetes.
We gratefully acknowledge the financial support by the Brazilian Council for
Scientific and Technological Development (CNPq).
References
Henquin JC: Triggering and amplifying pathways of regulation of
insulin secretion by glucose. Diabetes 2000;49:1751-1760.
Bliss CR, Sharp GW: Glucose-induced insulin release in islets of
young rats: Time-dependent potentiation and effects of 2-
bromostearate. Am J Physiol 1992;263:E890-896.
Grill V, Asplund K, Hellerstrom C, Cerasi E: Decreased cyclic amp
and insulin response to glucose in isolated islets of neonatal rats.
Diabetes 1975;24:746-752.
Reaven EP, Gold G, Reaven GM: Effect of age on glucose-stimulated
insulin release by the beta-cell of the rat. J Clin Invest 1979;64:591-
Reaven GM, Reaven PD: Effect of age on glucose oxidation by
isolated rat islets. Diabetologia 1980;18:69-71.
Waterland RA, Garza C: Early postnatal nutrition determines adult
pancreatic glucose-responsive insulin secretion and islet gene
expression in rats. J Nutr 2002;132:357-364.
Launer LJ: Diabetes and brain aging: Epidemiologic evidence. Curr
Diab Rep 2005;5:59-63.
Malaisse WJ, Malaisse-Lagae F, Coleman DL: Insulin secretion in
experimental obesity. Metabolism 1968;17:802-807.
Beck P, Koumans JH, Winterling CA, Stein MF, Daughaday WH,
Kipnis DM: Studies of insulin and growth hormone secretion in
human obesity. J Lab Clin Med 1964;64:654-667.
Barzilai N, Rossetti L: Relationship between changes in body
composition and insulin responsiveness in models of the aging rat. Am
J Physiol 1995;269:E591-597.
Heywood WE, Mian N, Milla PJ, Lindley KJ: Programming of
defective rat pancreatic beta-cell function in offspring from mothers
fed a low-protein diet during gestation and the suckling periods. Clin
Sci (Lond) 2004;107:37-45.
Martins PA, Sawaya AL: Evidence for impaired insulin production
and higher sensitivity in stunted children living in slums. Br J Nutr
Hirata AE, Alvarez-Rojas F, Carvalheira JB, Carvalho CR, Dolnikoff
MS, Abdalla Saad MJ: Modulation of ir/ptp1b interaction and
downstream signaling in insulin sensitive tissues of msg-rats. Life Sci
Balbo SL, Bonfleur ML, Carneiro EM, Amaral ME, Filiputti E,
Mathias PC: Parasympathetic activity changes insulin response to
glucose and neurotransmitters. Diabetes Metab 2002;28:3S13-17;
discussion 13S108-112.
Moreno G, Perello M, Camihort G, Luna G, Console G, Gaillard RC,
Spinedi E: Impact of transient correction of increased adrenocortical
activity in hypothalamo-damaged, hyperadipose female rats. Int J
Obes (Lond) 2006;30:73-82.
Bernardis LL, Patterson BD: Correlation between "lee index" and
carcass fat content in weanling and adults female rats with
hypothalamic lesions. J. Endocrinol. 1968;40:527-528.
Gravena C, Mathias PC, Ashcroft SJ: Acute effects of fatty acids on
insulin secretion from rat and human islets of langerhans. J
Endocrinol 2002;173:73-80.
Moura AS, Carpinelli AR, Barbosa FB, Gravena C, Mathias PC:
Undernutrition during early lactation as an alternative model to study
the onset of diabetes mellitus type ii. Res Commun Mol Pathol
Pharmacol 1996;92:73-84.
Blair SC, Caterson ID, Cooney GJ: Insulin response to an intravenous
glucose load during development of obesity in gold thioglucose-
injected mice. Diabetes 1993;42:1153-1158.
Barzilai N, Banerjee S, Hawkins M, Chang CJ, Chen W, Rossetti L:
The effect of age-dependent increase in fat mass on peripheral insulin
action is saturable. J Gerontol A Biol Sci Med Sci 1998;53:B141-146.
Catalano KJ, Bergman RN, Ader M: Increased susceptibility to
insulin resistance associated with abdominal obesity in aging rats.
Obes Res 2005;13:11-20.
Shafrir E: Animal models of non-insulin-dependent diabetes. Diabetes
Metab Rev 1992;8:179-208.
Marmo MR, Dolnikoff MS, Kettelhut IC, Matsushita DM, Hell NS,
Lima FB: Neonatal monosodium glutamate treatment increases
epididymal adipose tissue sensitivity to insulin in three-month old
rats. Braz J Med Biol Res 1994;27:1249-1253.
Macho L, Fickova M, Jezova, Zorad S: Late effects of postnatal
administration of monosodium glutamate on insulin action in adult
rats. Physiol Res 2000;49 Suppl 1:S79-85.
Balbo SL, Mathias PC, Bonfleur ML, Alves HF, Siroti FJ, Monteiro
OG, Ribeiro FB, Souza AC: Vagotomy reduces obesity in msg-treated
rats. Res Commun Mol Pathol Pharmacol 2000;108:291-296.
Dolnikoff M, Martin-Hidalgo A, Machado UF, Lima FB, Herrera E:
Decreased lipolysis and enhanced glycerol and glucose utilization by
adipose tissue prior to development of obesity in monosodium
glutamate (msg) treated-rats. Int J Obes Relat Metab Disord
Mozes S, Sefcikova Z, Lenhardt L, Racek L: Obesity and changes of
alkaline phosphatase activity in the small intestine of 40- and 80-day-
old rats subjected to early postnatal overfeeding or monosodium
glutamate. Physiol Res 2004;53:177-186.
Dawson R, Jr., Simpkins JW, Wallace DR: Age- and dose-dependent
effects of neonatal monosodium glutamate (msg) administration to
female rats. Neurotoxicol Teratol 1989;11:331-337.
Maiter D, Underwood LE, Martin JB, Koenig JI: Neonatal treatment
with monosodium glutamate: Effects of prolonged growth hormone
(gh)-releasing hormone deficiency on pulsatile gh secretion and
growth in female rats. Endocrinology 1991;128:1100-1106.
Kanarek RB, Meyers J, Meade RG, Mayer J: Juvenile-onset obesity
and deficits in caloric regulation in msg-treated rats. Pharmacol
Biochem Behav 1979;10:717-721.
Furnsinn C, Komjati M, Madsen OD, Schneider B, Waldhausl W:
Lifelong sequential changes in glucose tolerance and insulin secretion
in genetically obese zucker rats (fa/fa) fed a diabetogenic diet.
Endocrinology 1991;128:1093-1099.
Chang AM, Halter JB: Aging and insulin secretion. Am J Physiol
Endocrinol Metab 2003;284:E7-12.
Payne AH, Kelch RP, Murono EP, Kerlan JT: Hypothalamic,
pituitary and testicular function during sexual maturation of the male
rat. J Endocrinol 1977;72:17-26.
Ball GD, Huang TT, Gower BA, Cruz ML, Shaibi GQ, Weigensberg
MJ, Goran MI: Longitudinal changes in insulin sensitivity, insulin
secretion, and beta-cell function during puberty. J Pediatr
Hirata AE, Andrade IS, Vaskevicius P, Dolnikoff MS: Monosodium
glutamate (msg)-obese rats develop glucose intolerance and insulin
resistance to peripheral glucose uptake. Braz J Med Biol Res
Pujol A, Berthault MF, Picon L, Penicaud L: Regulation of glucose
homeostasis in pre-obese zucker rats before and after weaning. Int J
Obes 1991;15:505-511.
Inuwa IM, El Mardi AS: Correlation between volume fraction and
volume-weighted mean volume, and between total number and total
mass of islets in post-weaning and young wistar rats. J Anat
Montanya E, Nacher V, Biarnes M, Soler J: Linear correlation
between beta-cell mass and body weight throughout the lifespan in
lewis rats: Role of beta-cell hyperplasia and hypertrophy. Diabetes
Sako Y, Grill VE: Coupling of beta-cell desensitization by
hyperglycemia to excessive stimulation and circulating insulin in
glucose-infused rats. Diabetes 1990;39:1580-1583.
Ogneva V, Nikolov B: Changes in pancreatic islets in aging wistar and
zucker rats: A histochemical and ultrastructural morphometric study.
Mech Ageing Dev 1994;74:35-46.
Persson-Sjogren S, Lindstrom P: Effects of cholinergic m-receptor
agonists on insulin release in islets from obese and lean mice of
different ages: The importance of bicarbonate. Pancreas 2004;29:e90-
Fig. 1 Effect of development and MSG treatment on fatness, fasting plasma
glucose and insulin levels of the rats. Obesity was evaluated by Lee index
(Panel A) and weight of periepididymal fat (Panel B). Plasma glucose (Panel C)
and insulin (Panel D) concentrations were obtained after 16 hours fasting. Data
represent mean ± SEM. To both groups, in all ages were used n = 40 animals.
*p<0.05 compared to controls at same age. Letters represent differences in group
controls and MSG: a – p<0.05 at 30 days; b – p<0.05 at 60 days and c – p<0.05 at
Time (min)
Time (min)
Time (min)
l/l)
o
m 20
(n
lin 15
Time (min)
Time (min)
Time (min)
Fig. 2 Plasma glucose and insulin levels during ivGTT from controls and
MSG-rats.
Results represent mean ± SEM of glucose increment at 30 days (Panel A), 60
days (Panel B) and 90 days (Panel C). Increments of insulin are showed at 30
days (Panel D), 60 days (Panel E) and 90 days (Panel F). To both groups and ages
n = 8-10 animals were used. *p<0.05 to compare controls at same time and age.
Glycemia (mmol/l.min)
Insulinemia (pmol/l.min)
3.21 ± 0.47 b
4.96 ± 0.40 *
361.17 ± 18.12 c
473.83 ± 25.28* b c
60 days 4.49 ± 0.27 a 6.05 ± 0.46 *
700.17 ± 90.32 c
1681.67 ± 220.00* a c
90 days 3.98 ± 0.24
5.99 ± 0.14*
1861.67 ± 158.38 a b
5926.67 ± 326.00* a b
Tab. 1 Effect of development and MSG treatment on rats' glucose and insulin AUC
during all ivGTT.
Data represent mean ± SEM of glucose and insulin AUC in min during all ivGTT in
MSG – obese and control rats at 30, 60 and 90 days. N= 8-10 animals to both groups and
ages. *p<0.05 to compare to controls at the same age. Letters indicate intra group
differences : a – p<0.05 to 30-day-old; b – p<0.05 to 60-day-old; c – p<0.05 to 90-day-
Insulinogenic Index (∆I/∆G)
112.51 ± 27.51 c
95.53 ± 10.57 b c
155.93 ± 30.28 c
278.04 ± 22.97 * a c
467.76 ± 51.11a b
989.98 ± 51.38* a b
Tab. 2 Effect of development and MSG treatment on rats'insulinogenic index.
Data are mean ± SEM of insulinogenic index during ivGTT in obese – MSG and
control animals at 30, 60 and 90 days. N = 8 -10 animals were used in both groups and
ages. *p<0.05 compared to controls at the same age. Letters represent intra group
differences: a – p<0.05 to 30-day-old; b – p<0.05 to 60-day-old; c – p<0.05 to 90-day-
Glucose [mM]
Glucose [mM]
Glucose [mM]
Fig. 3 Effects of the different glucose concentrations on insulin secretion in isolated
pancreatic islets from control and MSG-rats .
Data represent mean ± SEM of insulin stimulated by glucose (2.8 – 20mM) in isolated
islets of MSG and control animals at 30 days (Panel A), 60 days (Panel B) and 90 days
(Panel C). At least 3 animals each group and age were used to isolate islets for 20
observations of insulin release induced by each glucose concentrations. *p<0.05 to
controls at same age and glucose concentration
Glucose [mM]
Glucose [mM]
Fig. 4 Effect of development on insulin secretion stimulated by glucose in
isolated islets.
Data are the same showed in the previous figure. To emphasize the effect of
development, secretion results are showing to isolated islets from control (Panel A) and
MSG-animals (Panel B) at 30, 60 and 90 days. Letters indicate differences of p<0.05 to:
a – 30-day-old; b – 60-day-old; c – 90-day-old.
• Body fats, circulating levels of insulin and peripheral resistance increase
simultaneously during development; all parameters are greatly enhanced by MSG
neonatal treatment.
• Secretory responses of pancreatic islets adjust themselves to the physiological state
and are more responsive in MSG rats in which resistance to insulin and fat
accumulation are higher.
• Development does not influence sensitiveness to glucose or to acetylcholine, its
main potentializer, but greatly modifies maximum secretory responses to the two
• Throughout their development pancreatic islets of MSG rats modify their secretory
control: initially an increase in sensitiveness to glucose occurs; afterwards, their
secretory capacity increases and, consequently, the normalization of sensitiveness to
glucose, when compared to lean rats.
• Glucose activity in K+ATP pathways remains unchanged in islets of MSG fat rats but
the amplifying effects of the secretor are absent.
• Acetylcholine effects on glucose-stimulated insulin secretion in pancreatic islets
also undergo changes throughout development but sensitiveness is preserved to the
detriment of maximum response to agonist.
• Pancreatic islets of fat MSG rats show similar characteristics in their response to
acetylcholine of islets of lean animals during development, but acetylcholine effects
decrease in all ages.
• Decrease in acetylcholine effect in MSG animals' islets may be attributed to a
reorganization and/or function of muscarinic receptors. This hypothesis is
corroborated by the inhibitory effect of acetylcholine mediated by subtype M2
muscarinic receptors.
• Functional adaptations of pancreatic islets of fat animals are not permanent and may
be reversed, as transplantation has shown.
artigo 2 publicado.pdf
artigo 4 publicado.pdf
Source: http://www.pbc.uem.br/grassiolli2006.pdf
REPOBLIKAN'I MADAGASIKARA Tanindrazana – Fahafahana – Fandrosoana COMMUNE URBAINE NOSY BE DISTRICT NOSY BE PROVINCE ANTSIRANANA I – Monographie b. De 1840 à 1896 c. De 1896 à 1960 d. De 1960 à nos jours 2. Situation géographique et délimitation administrative a. Situation géographique b. Organisation administrative 3. Les acteurs de développement
The influence of surface treatments on the service lives of concrete bridges SA Freitag SM Bruce Opus International Consultants Ltd, Lower Hutt NZ Transport Agency research report 403 ISBN 978-0-478-36421-7 (print) ISBN 978-0-478-36420-0 (electronic) ISSN 1173-3756 (print) ISSN 1173-3764 (electronic) NZ Transport Agency Private Bag 6995, Wellington 6141, New Zealand Telephone 64 4 894 5400; facsimile 64 4 894 6100 [email protected] www.nzta.govt.nz