Microsoft word - tamara jackson_trip report_v2.docx
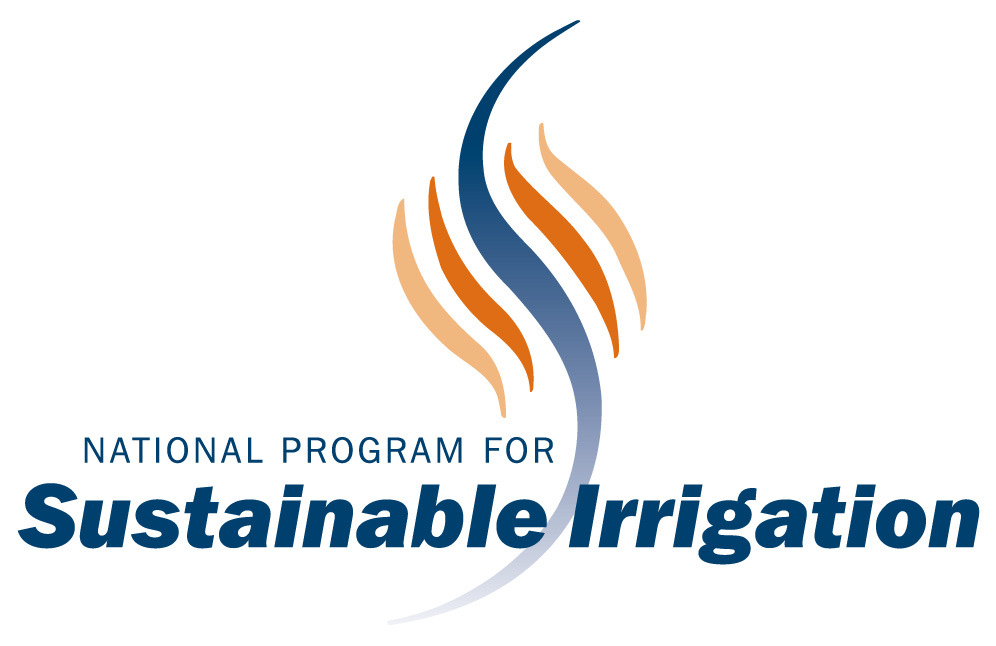
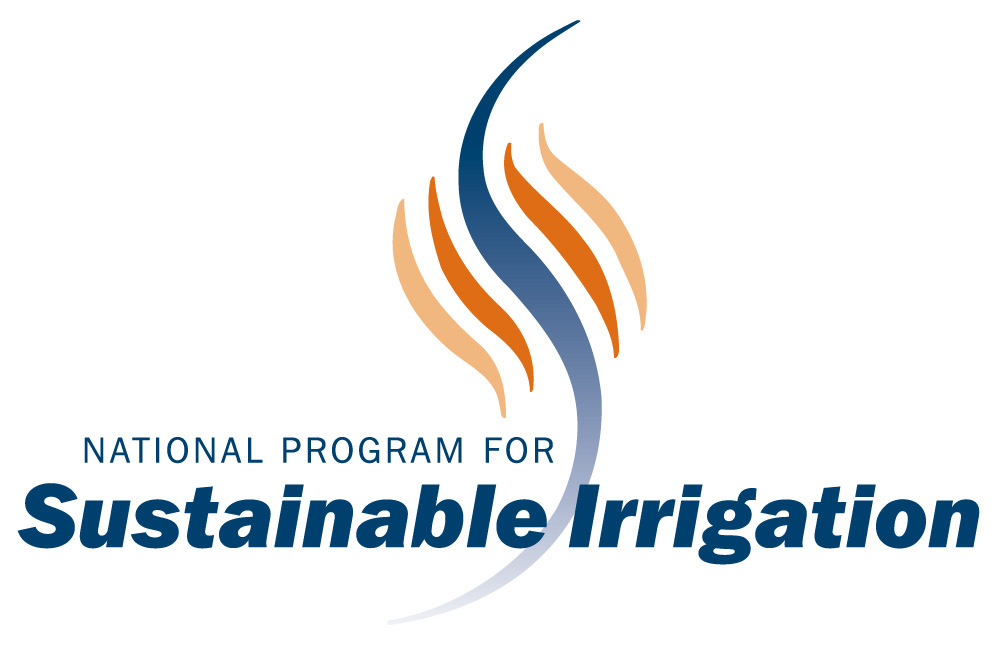
The water-en
e ergy-em
Tamara Jackson
IAL Travel Fellowship 2009
CONTENTS
Acknowledgements . 3
Background to study topic . 4
The Australian situation . 7
Irrigation industry . 10
Observations . 11
Agricultural energy consumption . 11
State of technology & importance of energy issues . 14
Irrigation industry . 16
Observations . 19
Current agricultural practices . 19
Irrigation research . 20
Technological advances . 21
United Kingdom . 25
Irrigation industry . 25
Observations . 27
Climate change . 27
Carbon accounting & carbon sequestration . 27
Water accounting . 29
Field visits . 31
Irrigation industry . 32
Tamara Jackson
IAL Travel Fellowship 2009
Observations . 34
Energy and carbon in agriculture – Professor David Pimentel . 34
Pump efficiency programs. 36
Water and renewable energy – the biofuel debate . 37
Water tensions in California . 38
Field visits . 40
Lessons learnt & opportunities for Australia. 43
Bibliography . 46
Tamara Jackson
IAL Travel Fellowship 2009
LIST OF FIGURES
Figure 1 Discussions with Dr Bilal Acar and Dr Ramazan Topak at Selcuk University, Konya.
. 13
Figure 2 Presenting at the Irrigation and energy use workshop at Nekim Kemal University, Tekirdag (left); and visiting an irrigated farm (right). 15
Figure 3 Plastic tunnels for tomato production in the Arava region (left), and a state of the art greenhouse in the Upper Galilee (right). 20
Figure 4 Energy use for different irrigation methods, including Low Pressure Drip (LPS). 23
Figure 5 Low Pressure Drip System at Kibbutz Yot Vita, near Eilat. 23
Figure 6 Centre pivot at Kibbutz Yot Vita, near Eilat. 24
Figure 7 Area of potatoes and vegetables irrigated, 2000 – 08 (DEFRA, 2009). 25
Figure 8 Licensed water abstracted for irrigation, 1995 – 2007 (DEFRA, 2009). 26
Figure 9 A farm sign in The Fens area; the symbols at the bottom of the sign are all different programs that this farm is accredited with. 30
Figure 10 A typical landscape in "The Fens". In summer, these drains are blocked and used to sub-irrigate the neighbouring fields. 31
Figure 11 Water source, 2003 and 2008 . 32
Figure 12 Energy expenses for irrigation pumps, 2008 (total 2.68 billion). 33
Figure 13 With Professor David Pimentel at Cornell University. 34
Figure 14 The California Aqueduct. 39
Figure 15 "Airjection" at Stamoules Produce. 41
Figure 16 Drip irrigation installed at Errotabere Ranch. 42
The topic of this travel fellowship was the water-energy-emissions nexus in irrigated
agriculture. This fellowship allowed me to meet with world class researchers working on
various aspects of this topic, extending my network of industry contacts. A key benefit was
the exposure to the latest research and ideas, which will provide an opportunity for these to
potentially be adapted to the Australian situation. Similar research carried out as part of my
PhD studies is pertinent to the major challenges facing irrigators both in Australia and
internationally. Given the current global focus on water and food shortages in conjunction
with a drive to reduce emissions, this fellowship provided a timely opportunity to explore the
links between these factors in irrigated agriculture.
The countries visited were Turkey, Israel, the UK and US. The irrigation industry in each of
these countries is very different in terms of scale, technology, legislation and state of
research. In Turkey I met with researchers from three universities, several of whom were
working on energy in irrigation for pumping. Discussions with Dr Bilal Acar and Dr Ramazan
Topak were useful in terms of understanding their approach to quantifying energy
In Israel, the highly technological state of irrigation and water resources management was
evident everywhere. I had the opportunity to travel from the relatively fertile north to the
extremely arid south of the country, witnessing throughout the abrupt changes in landscape
and methods of adaptation. The high input irrigation sector was explored in
nurseries/greenhouses, horticultural, viticultural and broad acre production systems.
In the UK, my experiences focussed on water and carbon accounting and climate change
impacts, where there is a lot of interest in these areas, both on the part of researchers but
also driven by consumer demand through supermarkets as well as government
The USA gave me the chance to discuss the issues surrounding biofuel development, as
well as to meet with several irrigators to explore their methods of coping as water availability
declines. A particular highlight was meeting with Professor David Pimentel at Cornell
University, who was the pioneer of research into energy in ecosystems and agriculture in the
1970s, and continues his research to this day.
This travel fellowship has allowed me to both contribute my ideas and learn from others
regarding the issues facing irrigated agriculture internationally, and to explore the potential
impacts of climate change on this vital sector of the agricultural industry. A better
understanding of these challenges will help with the development of strategies to adapt to
changes brought about by a changing climate and related policies.
This travel fellowship was funded by Irrigation Australia Limited and the National Program for
Sustainable Irrigation. Thanks go to these institutions for providing this opportunity. Support
was also provided by the International Centre of Water for Food Security at Charles Sturt
Particular thanks go to the following people for hosting me throughout my trip:
Dr Fatih Konukcu and staff at Nekim Kemal University, Tekirdag Ms Melis Sacan and Mr Emre Inalpulat, Cannakale Dr Duygu Kezmes and colleagues, Ankara University Dr Bilal Acar, Dr Ramazan Topak and colleagues at Selcuk University, Konya
Professor Avi Shaviv and staff at the Technion, Haifa Mr Dubi Raz and colleagues, Netafim
Dr Jerry Knox, Dr Keith Weatherhead, Dr Andre Daccache, Dr Tim Hess, Dr Ian Holman, and Dr Adrian Williams Cranfield University Professor Pete Smith, University of Aberdeen
Mr Mike Shannon and Mr Bob Farvad, USDA ARS Professor David Pimentel, Cornell University Mr Mike Wade, California Farm Water Coalition Mr Jose Ramirez, Manager, City of Firebaugh Dr David Zoldoske, Center for Irrigation Technology, California State University Mr Chuck Dees, Elena Stefanopoulos, Stamoules Produce Mr Dan Errotabere, Errotabere Ranches Mr Bill Taube, Wheeler Ridge-Maricopa Water Storage District
1 Background to study topic
Water and energy are critical drivers for life as well as agricultural production. Agricultural
processes combine water and energy resources to produce food and fibre crops for human
and animal consumption. The current level of global growth and development achieved can
be attributed to the quality and quantity of water and energy being consumed (Hellegers et
al., 2008). However, the consumption of these resources is not without consequence. It is
therefore imperative that we consider the sustainability of these precious but limited
resources in relation to agriculture, if we are to continue to provide food for the global
population and to achieve the required gains in production necessary in the future.
The world is currently facing a number of social and environmental issues. Socially, the
expanding population and improved standards of living are resulting in increased demands
for food and water in terms of both quantity and quality (Oenema et al., 2001). The world's
population is expected to reach eight billion by the year 2025, which is projected to require
an additional water supply of 15-20% for adequate agricultural production (Hamdy et al.,
2003). Environmentally, concerns include competing demand for water and the impacts of
climate change due to global warming, attributed to anthropogenic greenhouse gas (GHG)
emissions. It is inevitable that technology can and will play an important part in reaching a
solution to these problems; however, care must be taken to balance the benefits of any new
systems implemented with potential costs in terms of changes in energy consumption and
therefore potentially higher contributions to GHG emissions from agriculture.
Given that irrigated agriculture can be doubly as productive as rainfed agricultural land
(Entry et al., 2002), it can contribute greatly to increases in food production and is therefore
a vital part of world agriculture. Irrigation consumes 71% of the world's freshwater supply
(Zehnder et al., 2003), and increasing competition for freshwater resources from industry
and the environment means that the required increases in food production will need to come
from the same or a reduced quantity of water than is currently used. Water use efficiency
(WUE) in agriculture is currently low, with over 50% of water diverted for irrigation lost from
the system (Hamdy et al., 2003). This means that there is great scope to meet the growing
demand for water from water savings in agriculture. It has been identified that for many
irrigation systems, up to 55% of water that arrives at a farm is lost through poorly maintained
and designed distribution systems and inefficient field application practices (Zehnder et al.,
2003). Thus, changes to on-farm practices which increase WUE have the potential for water
savings that can meet the growing demand for water.
Much research into increasing WUE and therefore generating water savings focuses on the
conversion of gravity-fed irrigation systems to pressurised systems (Zehnder et al., 2003;
Lal, 2004; Pratt Water, 2004). Whilst the implementation of pressurised systems may be a
viable option due to the potential increase in water use efficiency (Phocaides, 2001), it must
be remembered that irrigation is a primary consumer of energy on farms (Naylor, 1996), and
these conversions may increase on-farm energy consumption. Singh et al. (2002) found that
irrigation always consumed the largest proportion of on-farm energy in their case studies of
agricultural production in India's arid zone.
It is recognised that energy use is necessary for increasing agricultural productivity and
improving food security (Sayin et al., 2005); indeed, intensification of fossil energy use has
been associated with an increase in agricultural productivity during recent decades (Conforti
and Giampetrio, 1997). The assessment of water and energy consumption in agricultural
production is vital to identify the major sources of wastage, and to determine strategies for
best allocating scarce resources to maintain production. The need to assess the resource
consumption and impacts of irrigation systems in light of current issues mean that there are
several factors that must be considered. Water use efficiency is currently a common
measure of the performance of irrigation systems; however it is important to assess these
systems in a broader sense, incorporating technical, economic and environmental aspects.
The trade-offs between these factors must be addressed, and the level of affordability of a
society determined in order to achieve the most appropriate balance between water and
energy consumption and the environmental consequences associated with GHG emissions.
Energy use by the agricultural sector depends on the amount of arable land available and
the level of mechanisation (Ozkan et al., 2004). Inanimate energy use is likely to be greater
in developed countries which have a higher level of mechanisation than developing
countries. Thus, the impacts of energy consumption are likely to be higher from developed
countries until or unless more technologically advanced options are adopted in developing
countries. If this occurs, the impacts of increased energy consumption will be of concern.
The inclination towards higher input systems and current levels of energy inefficiency in
agricultural systems may be due to low energy costs. A similar trend has been experienced
in regard to water consumption. However, this scenario is changing under current conditions,
where energy costs are becoming a primary consideration for producers, and one of the
fastest growing cost inputs (Chen et al., 2008).
There are many studies that seek to quantify the energy consumption associated with crop
production in various countries (Pimentel et al., 2002; Barber, 2004; Canakci et al., 2005;
Tzilivakis et al., 2005; Chamsing et al., 2006; Chaudhary et al., 2006; Hatirli et al., 2006;
Erdal et al., 2007; Esungen et al., 2007; Ozkan et al., 2007; Singh et al., 2007; Khan et
al., 2009; Jackson et al., 2010). While irrigation energy use is included where appropriate by
these studies, the integration of water and energy consumption is not considered, with the
exception of Khan et al. and Jackson et al.
Both Hodges et al. (1994) and Lal (2004) found that approximately 23% of direct energy use
for crop production in the US was used for on-farm pumping, indicating that irrigation can be
a significant energy cost for primary producers. Where a groundwater source is used for
irrigation, the use of pressurised micro-irrigation systems can decrease energy consumption
where reduced operating pressures and pumping volumes are experienced (Hodges et al.,
1994; Srivastava et al., 2003). This is an important factor that needs further exploration.
Given that water and nutrients are the principle limiting factors for crop production, the
supply of these inputs in cropping systems allows large gains in production (Martin et al.,
2006). However they can also represent large energy inputs into a system; therefore
methods that allow a reduction in the use of these inputs will reduce energy use by
agricultural systems. It is imperative that the criteria used to assess the sustainability of
agricultural systems reflect the issues of the time (Lal, 2004). Thus, a combined assessment
of water, energy and environmental impacts is essential in order that improvements to
systems on a global scale that will result in efficient and environmentally sound production
systems (Ozkan et al., 2004).
1.1 The Australian situation
South-eastern Australia is currently experiencing the worst drought since European
settlement, which has reduced agricultural outputs and resulted in water stress in many
regions. Irrigation allocations have been highly variable during the past ten years, with 0%
allocation in many regions. Climate forecasts for 2030 relative to the climate of 1990 predict
a reduction in annual rainfall by between 2-5% in the south of the continent, and an increase
in average temperature in southern Australia of approximately 1.0ºC, with warming of around
0.7-0.9ºC in coastal areas and 1-1.2ºC inland (CSIRO, 2007). These conditions are likely to
reduce water availability and increase water requirements by agricultural crops. Policy
makers and landholders will therefore need to adapt their policies and farming practices to
these new conditions.
Current government policy in Australia supports the modernisation of irrigation systems, with
the aim of generating water savings of over 2,500 GL per year within the Murray-Darling
Basin, where 85% of Australia's irrigation takes place (Department of the Environment,
Water, Heritage and the Arts, 2008). It is reasonable to assume that irrigators will be
encouraged to adopt pressurised irrigation systems in order to generate significant water
savings, despite the fact that there are few details regarding the specific methods for
modernisation. This will have far-reaching implications for water and energy consumption, as
well as environmental impacts.
There is a wealth of information regarding crop water use under Australian conditions, but
energy use and GHG emissions in agriculture have been less thoroughly researched.
Examples of energy consumption are limited in scope, concentrating on only energy use by
irrigation systems (Amaya, 2000; Michael Young and Associates, 2005) or direct energy
inputs (Chen and Baillie, 2007; Chen et al., 2008). The work done by Khan et al. (2009) and
Jackson et al. (2010) as previously described, are the only exception. Greenhouse gas
calculators that have been developed for agricultural production in the Australian context are
a good base to start, but more information is required. Thus there is a need to explore the
current state of research into water and energy consumption and GHG emissions associated
with crop production.
2 Itinerary
Country Date
Activities
Institution
Irrigation and energy use workshop
with university staff members, State
Hydraulic Department staff members,
Water resources & irirgation in Turkey
South Eastern Anatolian Project (GAP)
iii) State Hydraulic Works: irrigation and
energy infrastuructures in Turkey and particularly in Thrace Region
iv) Ipsala State Hydraulic Works will
present on rice irrigation practices (50% of Turkey's rice production is from this region), water resources, energy use, irrigation methods, etc.
Field visit to local irrigated farm, agricultural machinery factory
Meeting with university staff and
February presentation to staff and final year
Agricultural engineering students
Agricultural Faculty,
Meetings with university staff and
discussions on energy use in irrigation
Department, Agricultural Faculty,
University, Konya
Meetings with university staff in the The Technion,
faculty of Civil and Environmental Haifa
energy use in irrigation
Visit to BaHa'ai Gardens
Field visit in the Western Galilee i) Efficient use of water and fertilizers
in greenhouses (reverse osmosis, water and nutrient recycle, rain water collection, irrigation water treatment) - Shefer Nurseries, Kfar Bialik
ii) Avocado tree irrigation monitoring
research, Kibutz Rosh Hanikra
iii) Management aspects of avocado
and banana (net houses, soil mulching, irrigation and salinity field monitoring, water qualities and more), Koren Valley, Western Galilee.
February • Centre for Desert Research, Ben
Gurion University
Central and Northern Arava Research and Development – Greenhouse vegetable research station
Kibbutz Yot Vitah
SDA Spice – herb/spice producers & manufacturers
Banana/Mango plantations (Galilee)
Kibbutz Yifkah – Netafim training and research centre and factory (drippers, lay flat pipes – low pressure)
1st – 3rd • Presentation to Masters students
(Advanced Irrigation)
Dr Tim Hess (Reader in Water Management) – Water footprinting
Dr Ian Holman (Senior Lecturer) – Impacts of climate change
Dr Adrian Williams (Principal Research Fellow in Operational Research in Natural Resources) – Carbon footprinting
Visit to The Fens region – irrigation and drainage
Meeting with Professor Pete Smith,
University of Aberdeen
Discussion with Mike Shannon, Bob
Discussion with Professor David
Cornell University
California Farm Water Coalition/
Agricultural Water Management Council
Center for Irrigation Technology,
California State University, Fresno (Dr. David Zoldoske)
City of Firebaugh (Jose Ramirez, City Manager)
Stamoules Produce (Chuck Dees, Manager)
Errotabere Farms (Dan Errotabere)
Wheeler Ridge-Maricopa Water Storage District (Bill Taube, General Manager)
3.1 Irrigation industry
Turkey is unique in the world market, in that it is one of the few countries that are self-
sufficient in food production. The agricultural sector has been Turkey's largest sector in
terms of employment, and a major contributor to the country's Gross National Product,
although by 2007 this had declined to 11%. Almost 20% of Turkey's arable land area of 28
million hectares is currently irrigated (5.28 million hectares), and there is scope to further
irrigate 3.22 million hectares. Turkey's water activities have been comprehensively planned
since the 1950s. Currently, water management policies are directed towards satisfying the
growing demand for domestic water supply, achieving food security, generation of energy
and environmental conservation. Integrated regional development projects are an important
aspect of Turkey's water resource management, including the Southeastern Anatolia Project
(GAP), Eastern Anatolia Project (DAP) and Konya Plain Project (KOP).
Irrigation accounts for 74% (34 billion m3) of total surface water use; this proportion is
expected to decline to 64% (72 billion m3) by 2023. Most surface irrigation networks are
operated and maintained by Water User Organisations. In addition to this, groundwater use
by irrigation is 55% of the total allocation (6.77 billion m3). Groundwater irrigation is provided
by irrigation cooperatives, and all irrigation schemes are constructed by the government.
Investment costs are refunded within 15 years by farmers, free of interest. Groundwater is
allocated to farmers free of charge.
Major irrigated crop production in recent years includes maize, cereals, cotton, fruit and
vegetables. Approximately 92% of irrigation is carried out using surface irrigation methods
such as furrow, with the remainder using drip and sprinkler. The State Hydraulic Works
department identifies that gravity irrigation investment is cheaper than pumping irrigation
investments, and also specifies the financial burden of pumping costs for farmers as a
problem; thus gravity irrigation is the preferred option.
Key water resource issues in Turkey include:
The threat of water scarcity due to climate change
Extreme weather events such as droughts and floods
Improvement of water infrastructure and irrigation facilities
Integrated water and energy management for sustainable development – the need to
invest in hydropower
Transboundary water management – transboundary waters constitute 40% of Turkey's
water potential.
This information is taken from the Turkey Water Report (General Directorate of State
Hydraulic Works, 2009a) and the Water and DSI report (General Directorate of State
Hydraulic Works, 2009b).
3.2 Observations
3.2.1 Agricultural energy consumption
3.2.1.1 Previous studies
One of the motivations for selecting Turkey as a country to visit was several publications I
had read regarding agricultural energy consumption, at the farm and country level, including
the following publications:
Canakci, M., Topakci, M., Akinci, I., & Ozmerzi, A. (2005). Energy Use Pattern of
Some Field Crops and Vegetable Production: Case Study for Antalya Region,
Turkey. Energy Conversion and Management, 46(4), 655-666.
Erdal, G., Esungun, K., Erdal, H., & Gunduz, O. (2007). Energy use and economical
analysis of sugar beet production in Tokat province of Turkey. Energy, 32, 35-41.
Esungun, K., Erdal, G., Gunduz, O., & Erdal, H. (2007). An economic analysis and
energy use in stake-tomato production in Tokat province of Turkey. Renewable
Energy, 32, 1873-1881.
Hatirli, S. A., Ozkan, B., & Fert, C. (2006). Energy inputs and crop yield relationship
in greenhouse tomato production. Renewable Energy, 31, 427-438.
Ozkan, B., Akcaoz, H., & Fert, C. (2004). Energy input-output analysis in Turkish
agriculture. Renewable Energy, 29, 39-51.
Ozkan, B., Fert, C., & Karadeniz, C. F. (2007). Energy and cost analysis for
greenhouse and open-field grape production. Energy, 32, 1500-1504.
Sayin, C., Mencet, M. N., & Ozkan, B. (2005). Assessing of energy policies based on
Turkish agriculture: current status and some implications. Energy Policy, 33, 2361-
Unfortunately none of the researchers were available for me to visit. However, discussions
with Dr Bilal Hussein and Dr Ramazan Topak from Selcuk University, who are also working
in this area, were fruitful.
3.2.1.2 Current research into energy in irrigation
Dr Ramazan Topak from Selcuk University has been undertaking a large study of direct
energy use in irrigation in the Konya closed basin area. The Konya closed basin is 14% of
the total cultivated area of Turkey; it is also the driest area in Turkey. The water potential of
this region is 2.5% of Turkey's total potential water supply. Thus, there is a high cultivated
area but low water availability. Average rainfall is 323 mm per year, with an autumn/winter
rainfall dominant pattern. Rainfall is low during the production period. Winter cereals are
exposed to agricultural drought and must be irrigated. All arable land in the area is suitable
for irrigation. There is currently 550 000 ha irrigated in the region. The small average farm
size of 5 ha is a problem; however water availability is the biggest issue facing the region.
Sprinkler irrigation is the most common irrigation method. Field studies show that application
efficiency for sprinkler irrigation systems is 83%. The University projects that 80% of
tubewells used are associated with sprinkler systems. There are some surface irrigation
systems, as well as some drip on vineyards, orchards and recently introduced into field
crops. The average total efficiency is 73%.
The importance of direct energy consumption and the motivation to study this is due to
several factors, including the critical nature of irrigation in this region, a high proportion of
direct energy consumption and high diesel prices. As rainfall is virtually non-existent during
the crop production period, no irrigation means no yield. Direct energy consumption is very
high compared to indirect energy consumption, and up to 65% of total energy inputs into
crop production are associated with irrigation. Direct energy costs are expensive due to the
highest diesel prices in Turkey. Electricity costs are lower than diesel (a trend in most areas
of the world currently). Much of the work completed has looked at direct energy costs at the
field scale under farmer's conditions.
Dr Topak's study compared different irrigation regimes: 25%, 50%, 75% and full irrigation.
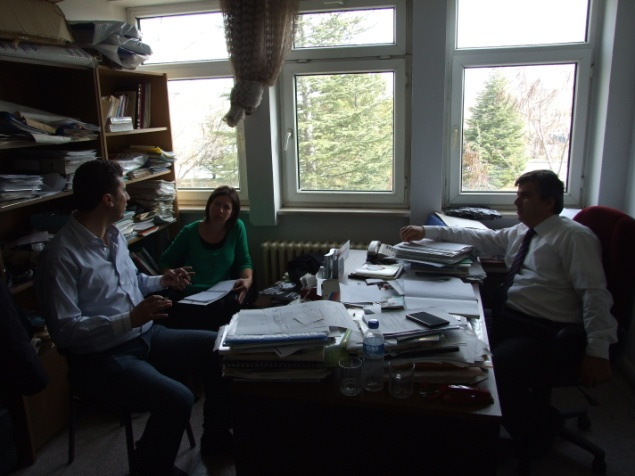
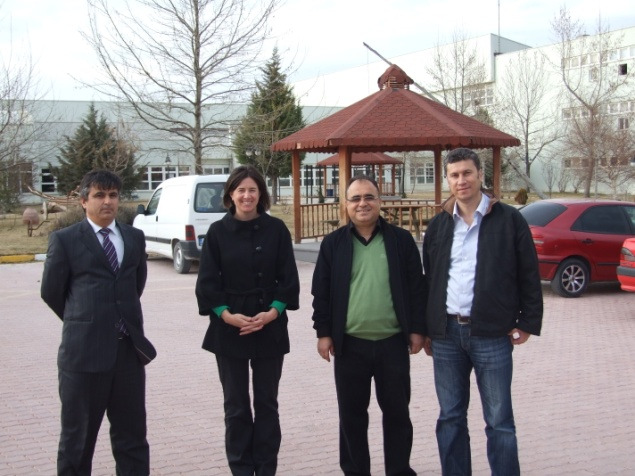
The results from the study show that for full irrigation using a drip system the energy
consumption is 52,000 MJ/ha, with irrigation accounting for 37 000 MJ/ha. There are
opportunities to reduce energy use with pressurised systems if the correct operating
pressure is selected, and also by controlling water application, since over irrigation leads to
higher energy consumption. The solution to this is irrigation scheduling and proper
We discussed the problem of upscaling of local results due to high variability. Dr Topak's aim
is to analyse the water and energy efficiency at the basin level (Konya), however the
researchers stated that there was very little/no variability among field/cereal crop production
throughout the region. There may be a problem with vegetable crops, but they generally
exclude these from calculations. When I asked about the high variability that I found during
my study (see Jackson et al., 2010) and how they would account for this, Dr Topak said that
to be more accurate, more information must be known about a region when there is high
variability. This is problematic in conditions where data collection is expensive. In their
experience they feel that surveys are not a good way to collect data – direct measurements
on farm are better, i.e. diesel consumption, average pressure etc. It is also essential to know
the system well in general.
Figure 1 Discussions with Dr Bilal Acar and Dr Ramazan Topak at Selcuk University, Konya.
3.2.2 State of technology & importance of energy issues
For farmers and government employees, energy consumption is primarily being considered
in terms of cost rather than physical energy use, or the impacts of this. In one way, this is a
positive as it brings energy consumption to a common unit of understanding for farmers,
state employees and researchers. In many of the projects that were presented, energy costs
(in terms of pumping) were included. For example, in one trial comparing different irrigation
methods for sunflower and maize crops, the cost of pumping was incorporated – and found
that costs more than doubled with the use of drip. In another project, the costs of pumping
using different water sources were compared. This estimated the cost of using groundwater
(600 TL/ha), surface water (150 TL/ha) and small earth dams - local systems with small
pumping systems - (50 TL/ha). Small, local systems were clearly optimal in terms of cost of
Typically in any research, it is common to concentrate on the most limiting factors; in Turkey,
this is usually water, but where this is not restricted, fertiliser is a major cost to farmers. One
staff member from Ankara University described a fertiliser trial where they had shown that
farmers can reduce nitrogen by 25% and phosphorous by 50% and still maintain yields.
From an energy perspective, this outcome also results in reducing energy inputs, as nitrogen
fertiliser is a major energy input.
Another joint project being undertaken in the Thrace region between Turkey, Bulgaria and
Greece is a scoping study to look at options for optimising water application; this also
considers energy costs of the different options studied. The next step is to look at
implementing some of the recommendations in these regions.
In a discussion at the workshop held at Nekim Kemal University, one of the presentations
showed that the difference in net revenue for a sunflower crop using 0% and 100% irrigation
was almost zero, but yield is almost double. The question was raised, why irrigate? The
predominant view was that Turkey and the irrigation industry benefits in terms of revenue
and increased food production, despite the loss of water and other possible unintended
environmental problems. This again raises the question of irrigation in broader terms than
just inputs and outputs.
Modernisation in terms of installation of pressurised systems and electricity costs of pumping
are supported by the government. The government subsidises 50% of the cost of
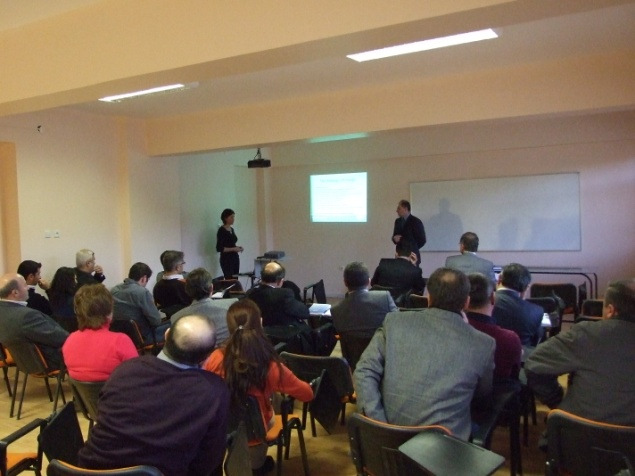
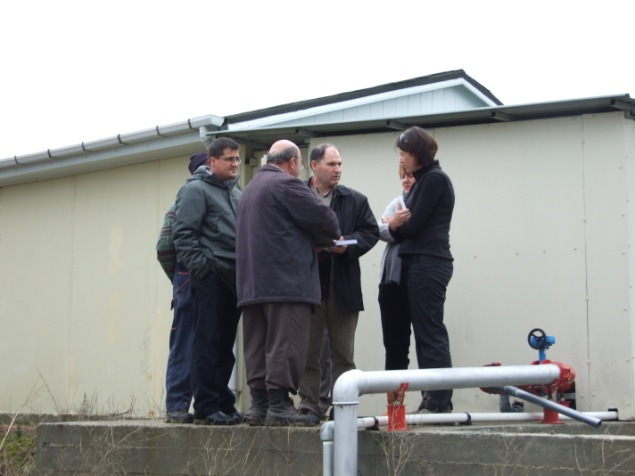
pressurised systems. Electricity for pumping is also subsidised (60% of full consumer price).
The farms visited showed highly modernised drip irrigation systems for horticulture.
However, according to local researchers, in practice in many cases, there is a lack of
technical support and energy costs become a problem for farmers, and often they revert
back to using surface systems because of these issues.
Most of Turkey's irrigation is undertaken using gravity fed systems, although sprinkler and
drip are also used. Manufacturers of a portable Hose Reel Irrigation System with the
capacity to irrigate up to 5 hectares at once stated that farmers react quickly to the adoption
of this technology. More units are sold in a dry year, probably due to the portable nature of
these machines, and the relatively low cost (up to $25,000).
In discussing the importance of energy costs to farmers, it was mentioned that while they are
generally important, fertiliser and other costs are more important. The biggest problem facing
farmers is uncertainty in market prices, and the difficulty in covering costs of production.
None of the institutions spoken to were considering emissions from agriculture, although
some expressed the desire to do so. This is an emerging area in Turkey, and there was
acknowledgment from researchers that this will get more attention in years to come.
Figure 2 Presenting at the Irrigation and energy use workshop at Nekim Kemal University,
Tekirdag (left); and visiting an irrigated farm (right).
4.1 Irrigation industry
Irrigation is synonymous with Israel: scarce water resources and the pressure for food
security have driven Israel to have a highly technical, water efficient agricultural industry.
Israel's varied climatic, topographical and soil conditions (from sub-tropical in the north to
arid in the central and southern regions) make it possible to grow a wide range of agricultural
produce. At least 60% of the country is defined as arid or semi-arid. Rain only falls between
November and April, with uneven distribution of yearly precipitation, historically raging from
700mm in the north to less than 50mm in the south. About two-thirds of Israel's field crops
are grown on un-irrigated land. These rain-fed crops include wheat for grain and silage, hay,
legumes for seeds and safflower for oil. The remainder is summer crops such as cotton,
sunflowers, chickpeas, green peas, beans, corn, groundnuts and watermelon for seeds,
mostly irrigated. The amount of irrigated farmland is approximately 182,000 ha out of a total
area under cultivation of approximately 440,000 ha.
While agriculture today represents a low 2.4% of the Gross Domestic Product and four
percent of exports, agriculture has grown in absolute terms and played an important part in
Israel's economy for over five decades. Agricultural output in 2000 was worth about $3.3
billion, of which 20% was exported.
In some areas, such as the Arava and the Jordan Valleys, agriculture provides almost the
sole means of livelihood for the population. In 2000, approximately 72,000 people were
involved in farming, constituting about 1.7 percent of the country's workforce. In monetary
terms, Israel produces almost 70% of its food requirements. It imports sugar, coffee and
cocoa and much of its grains, oilseeds, meat and fish. However, these imports are partially
offset by exports of fresh agricultural produce and processed foods. Today, just under a fifth
of the income of Israel's farmers derives from the export of fresh produce, including such
products as flowers, avocados, vegetables that are out of season elsewhere, and certain
exotic fruits grown exclusively for export. In addition, some 442,000 tons of fruit and
vegetables – 16% percent of the entire crop - were sold to factories for processing and
Water saving has been a priority since the establishment of Israel; saving water, finding new
sources and making optimal use of scarce land still characterize the country's agriculture.
The country has eight major and several small-to-medium-sized companies producing
irrigation and filtration equipment. Israeli engineers and agriculturalists created the
revolutionary drip system, and continue to be world leaders in this technology today.
To overcome regional imbalances in water availability, most of the country's freshwater
sources have been joined into the National Water Carrier, an integrated network of pumping
stations, reservoirs, canals and pipelines which transfers water from the north, where most
of the sources are, to the agricultural areas of the semi-arid south.
The total average annual potential of renewable water amounts to some 1,800 GL, of which
about 95% is already exploited and used for domestic consumption and irrigation. About
80% of the water potential is in the north of the country and only 20% in the south. Israel's
main freshwater resources are: Lake Kinneret - the Sea of Galilee, the Coastal Aquifer -
along the coastal plain of the Mediterranean Sea, and the Mountain Aquifer - under the
central north-south (Carmel) mountain range. Additional smaller regional resources are
located in the Upper Galilee, Western Galilee, Beit Shean Valley, Jordan Valley, the Dead
Sea Rift, the Negev and the Arava. The long-term average quantity of replenishable water
from major water resources amounts to about 1,800 GL per year. Reclaimed wastewater
effluent, intercepted runoff and artificial recharge and desalination are also widely used
Table 1 Long term potential of renewable water
Resource
Replenishable
Quantities
(MCM/year)
The Coastal Aquifer
The Mountain Aquifer
Additional Regional Resources
Total Average
In addition to surface water resources, the national water company Mekorot has also
developed the following water supply options:
Deep wells at depths of 1,500 meters and more. Approximately 1,040 water wells
currently pump water throughout Israel, half of which are operated by Mekorot. Of
these, 150 wells are used for recharging purposes, while the remainder are used as
Rehabilitation of old wells through the use of state-of-the-art engineering
techniques, a process that costs 20% as much as drilling new wells.
Wastewater treatment and reclamation through advanced treatment methods.
Mekorot operates 8 water treatment plants with a daily flow of 500 ML (186 GL per
year). Following treatment, water is routed to one of nine effluent reclamation plants.
These plants desalinate the water and/or insert it into the soil for tertiary treatment
and to recharge the aquifer, generating 206 GL of effluent water per year.
Creating usable water through the desalination of brackish water, seawater and
treated wastewater. Mekorot operates 31 reverse osmosis (RO) and electro dialysis
(ED) desalination plants throughout Israel with a daily treatment capacity of nearly 1
GL. Through an ambitious building program, Mekorot intends to increase desalination
production by 2010 to nearly 200 GL per year, including 100 GL to be desalinated
from sea water, 77 GL from brackish water and 13 GL from secondary effluents and
Artificial recharge of groundwater at recharge plants located throughout the
country, including Shafdan, Shikma, Menashe rivers, Nachshonim and five reservoirs
south of the Dead Sea. Artificial recharge of Israel's Coastal Aquifer serves to restore
higher groundwater levels to counteract further intrusion of sea water and to
counteract withdrawals.
This information is taken from:
4.2 Observations
4.2.1 Current agricultural practices
Israeli agriculture is highly technologically advanced, precisely managed and reliant on
irrigation. In terms of access to water, irrigators do not own water rights; they have the
opportunity to use it indefinitely as long as it is used "productively". Access can be cut back if
it is not used. Water is used efficiently because it is scarce and expensive. Through
discussions with local industry leaders, they identified the biggest problems facing farmers in
Israel as being:
1. Lack of labour availability; internal/domestic labour is scarce, and most farm
labourers are recruited from Thailand – this makes farmers reliant on immigration
policy, which has been cut back lately;
2. Land and water availability; and
3. Market pressures.
Energy intensity in irrigation is not a matter of energy for pumping, since water is delivered
pressurised to farmers either from the national water grid (sourced from the Sea of Galilee),
recycled water or from groundwater. The government owns and distributes all water, and the
pressure it is delivered at varies but is mostly around 4 - 5 atmospheres (400 - 500 kPa).
Energy intensity is embedded in the highly mechanised nature of agricultural production. For
example, equipment used for irrigation (drip lines etc), use of plastics as mulch and covering,
heating and equipment for green/net houses (Figure 3). However there are strategies to
combat this, for example Netafim offers recycling of all driplines and then sells the next batch
to farmers at a cheaper rate. In terms of country scale emissions, agricultural emissions are
just 3% of the national total. Farmers are not thinking about controlling emissions or the
impacts of this, but at the ministry level they are. At this stage the discussion is around
targets in line with OECD/EU averages.
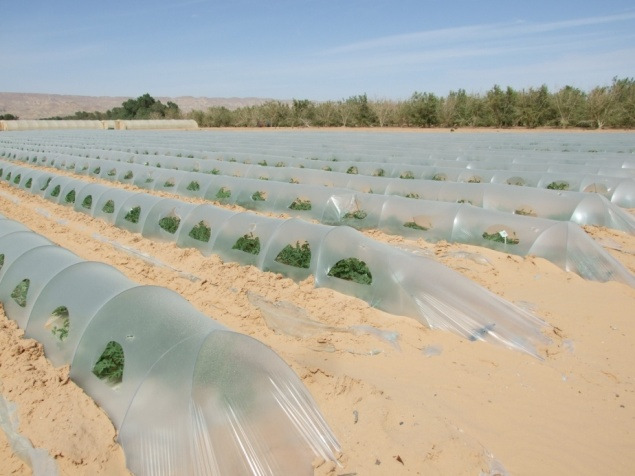
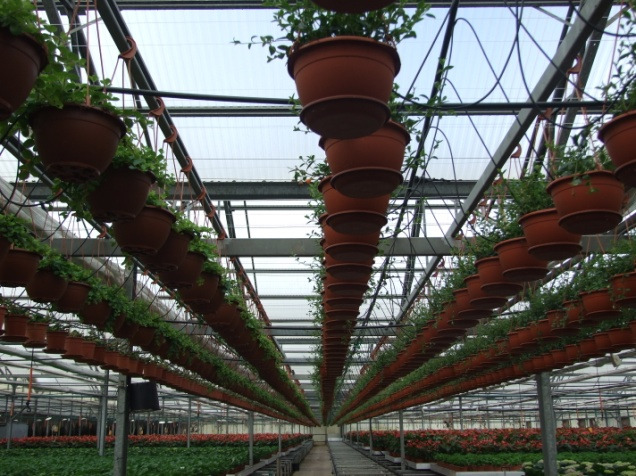
Figure 3 Plastic tunnels for tomato production in the Arava region (left), and a state of the art
greenhouse in the Upper Galilee (right).
Fertiliser appears to be closely monitored; for example, at one Kibbutz they were using
weekly petiole testing of potatoes to determine nitrogen fertiliser use. This was also used in
greenhouses visited. This offers an opportunity to reduce energy use by optimising uptake
by plants and preventing leaching; nitrogen fertiliser is often a major energy cost, due to the
energy intensive process by which it is manufactured, so any reduction in waste of nitrogen
fertiliser will also save energy. Emissions can also be reduced by optimally applying
nitrogen, through a reduction in nitrogen lost as nitrous oxide.
There is a need to look at energy productivity of Israeli agriculture in comparison to other
situations; while energy inputs appear to be high, yields are also high as. No studies of
energy use in Israeli agriculture could be found.
4.2.2 Irrigation research
At the Technion, I met with researchers in the Department of Engineering. No one is working
on irrigation directly, but they are involved with applied research which is linked indirectly to
irrigation, and see their role as more to advance knowledge of aspects of irrigation e.g. soil
physics, the movement and behaviour of fertiliser in soils, water movement in the root zone
etc. The researchers work closely with industry to solve problems using applied science to
understand systems. For example, with the increasing use of waste water, they have studied
the reactions of reclaimed wastewater on soil properties and plant interactions.
In terms of emissions, there is some research into emissions of N2O from streams, shallow
groundwater and from agricultural inputs. However, again this is at the process stage, for
example looking at how to evaluate sources and processes of N2O and which microbial
processes are affected. To do this, they are using isotopic based tools, merging soil physical
processes with data monitoring. There is also work comparing irrigation with wastewater and
fresh water, fertilisers/organic/non-organic production methods and different soils. To do this
they are using long pass Fourier Transfer Infra Red (FTIR) to quantify CO2/N2O emissions.
Other research is being undertaken using spatial and spectral data to identify salinity stress
in vegetation. It is hoped that this will result in appropriate irrigation depending on suitable
soil types, and improve irrigation scheduling/cycling. This research combined thermal data
with spatial and spectral data. It helped to identify areas of stress to isolate areas to further
investigate using ground based methods.
At the Central and Northern Arava Research and Development station, research into
greenhouse vegetable production is being undertaken. In this desert region, water resources
are extremely scarce (230 m3/day per farm) and farmers are also working with some of the
worst quality ground water in Israel (2.8 – 3.5 EC). Peppers, tomatoes, melons, vegetables
and cut flowers are produced in net or plastic greenhouses. These produce high quality fruit
and vegetables in winter for export to EU markets. In terms of research impacting on water
and energy management, trials include:
LED lights to reduce energy costs
Fertiliser type (Ammonium Nitrate vs Urea) to see if N quality has an impact on
yield productivity and product shelf life (this could also affect energy use)
Biological controls and spraying – thrips are resistant to pesticide use now – next
year farmers will use biological control as it is more effective (i.e. for peppers)
Trials being done to demonstrate the suitability of irrigating at night. Currently
farmers are only irrigating during the day and are reluctant to try night irrigation.
4.2.3 Technological advances
Most farms in Israel are very small and are dedicated to intensive nursery/vegetable
production. Broadacre crops tend to be grown on kibbutz or big farms. Often farm sizes are
now not big enough to earn a decent income.
A number of greenhouses and net houses were visited, including Shefer Nurseries in the
Western Galilee. This is an excellent example of efficient use of water and fertilisers in
ornamental greenhouses. Water application rates are approximately 1.5mm/day. In winter,
their water source is 100% from rainwater collected from the roof area of the greenhouses.
In summer, when rainfall is scarce, they use reverse osmosis to purify water, due to the
need for very high quality water for most varieties produced. Water quality of that water
delivered by the government has improved significantly in the last 1-2 years. Water prices
are a small part of the total annual budget as they are so efficient. In fact, the cooling system
uses more water than irrigation of plants. Water costs around 7-8 NIS/m3 (equivalent to
AU$2.25/m3) (pressurised and with all treatments, fertiliser etc included). The nursery is a
totally closed system, including water and nutrient recycling. Recycled water is treated using
biofiltration (80% effective) and thermal sterilisation (100% effective). Dr Anat Lowengart
(from the Ministry of Agriculture Extension Service) emphasised that Shefer Nurseries are a
good example of growers adopting new technology and using extension officers only when
they have a problem they cannot solve themselves
Avocado and banana research sites and productive farms were visited in the Western
Galilee. At Kibbutz Rosh Hanikra, Dr Amos Noor and Yaron Weissmark are conducting
avocado tree irrigation monitoring research. They are measuring eight water stress
indicators to determine their ability to withstand a reduction in water application. The project
is funded by grower industry groups. Similarly in the Koren Valley, trials into management of
avocado and banana using nets, soil mulching, irrigation, salinity field monitoring and
variable water quality are being undertaken. Bananas grown under netting have higher
yields, lower water use and better fruit quality. In the Galilee region, field scale water savings
are around 10% under nets (due to a more humid, subtropical climate). In the Jordan Valley
however, water savings are around 30%. Yields are improved in both regions.
Low pressure, low flow (0.6 l/hr) drip systems (LPS) developed by Netafim have the potential
to revolutionise water and energy management. These systems operate at between 2.5 –
8m head, and can be used in broad acre crops. LPS system (assumed operating pressure
5m) uses 13% energy compared to conventionally operated drip systems (assumed
operating pressure of 40m) (Figure 4 and Figure 5). One limitation of LPS is that they require
good quality water or energy for filtering, depending on water quality. Netafim are working on
the development of low/no energy filters, and have floating in line filters and other options.
However, it does mean that these systems will not be suitable everywhere – although it
gives options for reducing energy and water use in certain situations. Netafim's new low
pressure drip systems will not lead to saved energy costs within Israel due to the need in
many cases here to reduce pressure before these systems can be used, but they do offer a
chance to reduce water use, increase yields and crop quality and reduce fertiliser use.
CENTER PIVOT
Figure 4 Energy use for different irrigation methods, including Low Pressure Drip (LPS).
Figure 5 Low Pressure Drip System at Kibbutz Yot Vita, near Eilat.
One of the biggest Kibbutzim visited was Kibbutz Yot Vita, near Eilat. This Kibbutz has 300
members and comprises of 100 ha of intensive agriculture, a greenhouse, date orchard,
dairy and dairy processing factory. Most grain and fodder for the dairy is imported from the
north of the country. Most of the irrigation is undertaken using drip (including low pressure
drip), although they also have two centre pivots that use sewage water from Eilat, just used
to grow pasture for feed – simply because they need to use the water (Figure 6). Dairy
biogas is used to fuel the dairy operation.
Figure 6 Centre pivot at Kibbutz Yot Vita, near Eilat.
5 United Kingdom
5.1 Irrigation industry
The UK irrigation industry represents a very small proportion of total water use in the
country; water use for irrigated agriculture represents only 2% of all water use. Despite this
small proportion of water used, irrigated agriculture is economically very important,
contributing 20% of total crop value. Over the last 20 years, there have been significant
changes in the composition of the crops irrigated. The proportion of irrigation on pastures,
sugar beet and cereals has declined, and in contrast there has been an increase in irrigation
of high value crops, particularly potatoes and vegetables for human consumption. In 2005,
irrigated horticultural crops accounted for 74% of the total irrigated area, and 86% of the total
volume of irrigation water applied. Of these, potatoes and vegetables are the main irrigated
crops, accounting for 70% of water use; in 2008 around 106,000 ha of potatoes and a similar
area of vegetables were irrigated (Figure 7) (DEFRA, 2009).
Figure 7 Area of potatoes and vegetables irrigated, 2000 – 08 (DEFRA, 2009).
Agricultural abstractions have decreased by 48% during the period 1995 – 2007 (Figure 8).
Approximately 95% of irrigation water for agriculture is abstracted from surface (54%) and
ground (41%) water (DEFRA, 2009). Rainguns are the most commonly used irrigation
Figure 8 Licensed water abstracted for irrigation, 1995 – 2007 (DEFRA, 2009).
5.2 Observations
5.2.1 Climate change
At Cranfield University, I met with Dr Ian Holman, who is researching the integrated
assessment of the effects of future changes on natural resource management. These
include cross sectoral interactions and impacts, including climate and socio-economic
aspects of agriculture. Studies show that in the UK, agriculture is more sensitive to the
economic environment, and is relatively insensitive to climate. However, while this applies to
agriculture in general, irrigation is more sensitive as water resources are highly affected by
climate change. Part of the complexity in assessing irrigation and climate change is that
water availability and water allocation are determined by social values.
Dr Holman is interested in the impact of climate change on irrigated agriculture and how
adaptation will occur. There will need to be cash inputs to change infrastructure and
production systems. There is a need to manage landscapes gradually, and to plan for
transitions so that ecological processes can adapt to climate change. Maintaining species in
response to climate change may be untenable in some circumstances. To help adaptation,
non climate pressures should be reduced. Payments for Ecosystem Services are an option,
but is it right to create a market for something that is traditionally free?
5.2.2 Carbon accounting & carbon sequestration
Professor Pete Smith at the University of Aberdeen was the Convening Lead Author of the
Intergovernmental Panel on Climate Change (IPCC) Fourth Assessment Report, Mitigation
volume, Chapter 8 (2004 - 2007), and has published work on energy intensities and GHG
emission mitigation in agriculture. Irrigation included as a sequestration option due to the
opportunity to increase productivity. However, in most cases energy costs don't cancel out
improved organic matter and productivity. There are energy costs associated with applying
water, including GHG costs and embedded costs. Methane is probably a small component of
flooding (aside from rice production, which is often permanently flooded). However, N2O
emissions might be much better under drip (Kallenbach et al., 2010).
I discussed with Professor Smith his views on including or excluding agriculture from
emissions accounting/trading schemes. If it is left out, it's hard to give people incentives to
change, because it is not accounted for. But if it is kept in and used to ‘squeeze' producers, it
could bring the industry down. On a global level, there is a need for accounting, but it needs
to be managed carefully. Most developed countries already have country level agricultural
emissions. Accounting for bulk N is not accurate, as shown in accounting systems e.g.
precision farming. In his opinion, Australia has one of the best carbon accounting systems
(NCAS), the result of spending $12m per year from the early 2000s as opposed to £300K
pounds in the UK.
In terms of mitigating carbon emissions, Professor Smith thinks we need to look at the
biggest sources of emissions and try to get people to move towards lower emissions. Even
small changes can make a big difference; all the 0.5% add up. We need to ‘decarbonise' in
the next 20-25 years. Options include microgeneration of fuel – 5/7 emissions come from
fossil fuels. There is no time for nuclear development. Renewables are deployable now, and
big investments are needed now. Changing economics will force a change. In agriculture,
the reuse of mineral N and organic manures is essential, and can be forced by costs of N.
Boosting soil organic matter (carbon) has other benefits apart from carbon sequestration.
The problems with this are that it is time limited, reversible and easily lost. If we only have 20
years to make a change, carbon sequestration is not a wide scale solution – we cannot rely
on this. Carbon sequestration should be seen as a co-benefit rather than the driver. Farmers
are already being paid for carbon sequestration on the voluntary market – but it is not the
Dr Adrian Williams at Cranfield University undertakes agricultural systems based Life Cycle
Analysis, combining indicators for human health and resource consumption. He has worked
on projects at the national scale for the Department of Environment, Food and Rural Affairs
(DEFRA), looking at ten main field crops to compare those produced in the UK with imports
(Williams et al., 2008). We discussed methods of upscaling, and ways of breaking down
systems into biophysical components, i.e. soil texture, rainfall zones. Also need to look at a
range of activities, rather than just one aspect of a system. Variations in machinery use
(such as found in Australia) are not a problem in the UK – there is more homogenous use.
Any variation is taken from DEFRA's farm business survey. This starts with financial aspects
and incorporates energy interests – but with more of an aim at direct energy. Indirect energy
has now also been added. People use this information for carbon footprinting, but there is a
danger that this will miss finer points. There is a need to maintain diversity of understanding.
Dr Williams described a new project for the Committee on Climate Change regarding soil
carbon. This project explores consumption at the country level (UK), based on commodities
consumed, and the impacts of scenarios for reducing emissions within the UK. For example,
a large reduction in the production of meat products within the UK would free up arable land
in the UK, but would have impacts elsewhere. There is a need to examine the effects of this
– what would replace livestock production, and what would the impacts be? There are also
implications for land use change from imported crops. For example, there can be a shift in
international trade, i.e. organic soy is imported into the UK from China, but China then needs
to fulfil its soy requirements, and imports conventionally produced soy for their needs from
Brazil. Thus, there is not a net increase in organic production – and the carbon impacts of
this change in production due to trade influences. For example, organic, low energy food
products have a high land requirement, thereby increasing land pressures.
5.2.3 Water accounting
Dr Tim Hess at Cranfield University is conducting research into water footprinting. The main
aim of this is to refine water footprinting to take into account the impact of using water, not
just the amount of water used. That is, the impacts of water use in time, space and source.
Water footprinting takes into account the differences between blue (surface and groundwater
resources - irrigation), green (rainfall/soil moisture) and grey (polluted) water associated with
the production of any good or service. This approach gives a stress related water footprint,
and accounts for the impact of production from, for example, rainfed versus irrigated crops,
or crops produced in water scarce regions compared to those produced where water is
abundant. Producing a single number is meaningless unless we take into account the impact
of that water used, which is the advantage of the water footprinting approach.
When the water footprint of crops is examined, some studies take into account inputs, but
this works out to be 99% of water attributed to the growing process. There are major
differences for livestock systems – where using feed (irrigated or dryland) or grazing
systems (irrigated or dryland). Water footprinting is done from a top down approach on a
national scale, with flows on a national basis. In terms of accounting for variability, the
methods used assume the average method of production, multiplied by the quantity of crop
Ultimately, there are impacts on consumers from carbon and water footprinting that should
be considered. Supermarkets are using these indicators as a marketing tool to increase
profits; ultimately, supermarkets dictate what will be produced, and increasingly in the UK,
the methods by which they are produced. For example, up to 60% of the irrigated area in the
UK is scheduled using scientific (objective) approaches (Hess et al., 2009). Is this because
of the high value crops or because many supermarket QA processes require this as a
component of the process? Figure 9 shows an example of some of the supermarket
programs that farms are accredited with. People want carbon footprinting now, and they
want it with no errors – in reality, you get a value with an error band around it. A rating may
be better than a single number. There are potential legal constraints related to carbon
footprinting. Methods are needed for estimating the uncertainty related to these numbers.
Consumers range from informed to uninformed, but for a lot of people their consumer
preferences will still come down to price. Professor Pete Smith thinks that consumers are
becoming better educated, and carbon labelling is a benefit that will change behaviour as it
becomes more common and better understood.
Figure 9 A farm sign in The Fens area; the symbols at the bottom of the sign are all different
programs that this farm is accredited with.
5.3 Field visits
Along with Dr Jerry Knox, I visited Holme Fen, which is land that was reclaimed in the 1850s
(previously 2nd largest lake in UK). It is now highly productive agricultural land; soils have
very high organic matter and are uniform and very fertile. Major crops produced are field
crops (wheat etc.), potatoes, onions and spring onions. Crops give good yields and are of
good quality. Fields are drained in winter by a series of drains, which eventually drain via
large channels into the sea, where they are pumped at low tide. In summer, drains are
blocked and used to sub-irrigate fields (see Figure 10).
Figure 10 A typical landscape in "The Fens". In summer, these drains are blocked and used to
sub-irrigate the neighbouring fields.
6.1 Irrigation industry
In 2008 the US had a total irrigated area of 54.9 million acres (22.2 million hectares) in 2008,
an increase of 4.6% over the previous 5 years. The total quantity of water applied also
increased by 5%, up to 91.2 million acre feet (112,493.5 GL). The major irrigated states are
Nebraska, California, Texas, Arkansas and Idaho. There is excellent, comprehensive
information available from the 2008 Farm and Ranch Irrigation Survey (USDA, 2008),
including information on crop water use, irrigation method, decision making by irrigators and
energy use. Figure 11 shows the area and quantity of water applied from groundwater and
surface water. Groundwater is the dominant water source, accounting for over 53% of total
Figure 11 Water source, 2003 and 2008
Given that groundwater is the dominant irrigation water source, it is likely that the cost of
pumping will add significantly to costs. The above mentioned survey reports water expenses
per acre for groundwater as $60.9, and surface water as $36.13. This may explain why in
the 5 years between 2003 - 2008, almost 75,000 farms made changes to their irrigation
equipment or management practices that reduced energy use and/or conserved water.
Around 46% reported a reduction in energy costs and 59% a reduction in the amount of
water applied. Figure 12 shows that electricity is the biggest energy expense for irrigation
pumping, followed by diesel fuel and natural gas. The level of information available for
irrigation in the US is far greater than anything available in Australia, and indeed many other
Figure 12 Energy expenses for irrigation pumps, 2008 (total 2.68 billion)
6.2 Observations
6.2.1 Energy and carbon in agriculture – Professor David Pimentel
Professor David Pimentel from Cornell University is the pioneer of studies into energy in
ecosystems and agriculture. His career has developed from entomology, but he also
developed an interest in energy and biofuels. In 1968 he was part of a National Panel to
identify what would be the major problems facing agriculture over the next 25 years.
Professor Pimentel suggested energy, and all others on the panel disagreed. He then went
on to set up a Masters program in ecology and then in environmental policy. Along with his
Masters students, in 1970 he wrote the first paper on energy in agriculture. This took 3 years
to get published in Science, but when it did it coincided with the oil crisis of the 1970s, and
prompted further research into this subject which he continues today.
Figure 13 With Professor David Pimentel at Cornell University.
Professor Pimentel agrees that irrigation is essential, but questions whether all crops
currently irrigated necessary. We need to think about what types of crops should we be
irrigating and where. For example, lettuce is irrigated in California and then transported to
the east coast – this is intensive in terms of water and energy; for an energy output of 400
calories/head of lettuce, it requires 4000 calories for transport. In Nebraska, irrigated corn
uses three times as much energy as non-irrigated corn. Irrigating high yielding (in terms of
energy) grain crops may be suitable, or irrigating to save crops in times of drought to
maintain production. However, this would eliminate the possibility of investing in high tech
irrigation systems and complex water delivery and access systems if irrigation was going to
be spontaneous – it would not be economically feasible. Gravity fed irrigation systems still
have a place, given the energy intensity of pressurised systems.
Changing consumer preferences is also important in terms of the energy used for crop
production and storage. For example, in the US, most people eat twice as much as the body
requires (kcal), including twice as much protein which is energy intensive and emissions
intensive. There is a need to go back to locally produced, seasonal food.
Professor Pimentel undertook a 22 year study with the Rodale Institute, comparing organic
and conventional crops (corn and wheat). The conventional system was managed following
recommendations by Penn State University. At the end of 22 years, the organic system had
twice as much soil organic matter as the conventional system. In a drought year, the organic
system had 33% higher yields for corn and 50% for wheat. The energy use was 33% less in
the organic system, but 30% more labour was required. On average over the 22 years,
yields were the same for organic and conventional crops. Organic production offers the
potential to make our soils more resistant to drought.
Professor Pimentel sees genetically modified (GM) crops as an opportunity, but is opposed
to the types of GM research being undertaken at the moment (e.g. chemically tolerant
plants). There may be opportunities to reduce energy consumption through certain types of
GM – for example, he would like to see GM to make annual plants become perennial – in
order to reduce the energy required for soil cultivation, sowing and embedded in seeds.
However this would also have an effect on water use – what would the trade-offs be?
According to Professor Pimentel, looking to the future, we will run out of oil. This will
challenge the way we use water and produce crops. Pumping for irrigation on a wide scale
may not be viable; thus food production will move to more suitable climatic zones.
Transporting food and water will be a problem. This will cause major changes to agricultural
production, and consumer preferences will have to change. In short, water and energy are
the two items that will ‘upset' US agriculture.
Dr David Zoldoske is the Director of the Centre for Irrigation Technology at California State
University in Fresno (CSU-F). This centre is involved in several interesting projects related to
water, energy and emissions. In one experiment, they have used driplines to apply CO2 to a
crop canopy (tomatoes and peppers) in a plastic tunnel. Around 30-40 tonnes of CO2 are
applied over 45-60 days during the season after the canopy is established. The results are
good, with yield increases of 65% observed. In the year that the experiment was undertaken,
there were high levels of heat stress, therefore higher CO2 levels helped the plants cope with
this stress. In terms of the carbon balance, the CO2 used is a high quality by-product of
biomass use. No additional CO2 is being generated as they are using a waste product and
also sequestering more carbon due to increased yields.
6.2.2 Pump efficiency programs
CSU-F has developed and managed the Agricultural Pumping Efficiency Program. The
program is for agricultural water pumpers using electricity or natural gas, to help reduce
energy use and improve resource efficiency. The funding for the program comes from the
Public Goods Charge that is paid by ratepayers under the California Public Utilities
Commission. There are separate programs for electric and diesel pumpers due to the nature
of the funding secured to date. Under the electric pumping program, around 20,000 tests on
about 8,000 pumps have been performed. This covers a lot of the big motors and or pumps
with high hours. Big districts and city pumps already pay close attention to pump efficiency. 1
million kWh per month of new savings have been generated through improved pumping.
This offers huge potential for energy savings across the state.
Part of the driver for assessing diesel pumps is pollution reduction, in particular reductions in
N2O and particulate matter (less than 10 micro m). This reduction in pollution is due to
improved efficiency. So far there have been two pilot programs for diesel pumpers that have
been highly effective, but funding has ended. For the funding body, the interest has shifted
to fuel substitution, filtering etc. The results from the pilot programs show overall efficiencies
of 17-18%; this was able to be improved by 67%. This resulted in a reduction on average of
NOx emissions by 3.4 tonnes. It was also favourable in economics – with fuel bill halved in
many cases. Diesel pumps are estimated to make up around 10% of the state's irrigation
pumps, mainly used in isolated areas where access to electricity is poor.
6.2.3 Water and renewable energy – the biofuel debate
I met with Mike Shannon at the USDA in Beltsville, Maryland, who is the National Program
Leader for Water Availability and Watershed Management. As part of this department's
operations, it undertakes conservation assessments, looking at water availability and water
quality, and assessing risks to the natural resource base. A resource conservation
assessment is undertaken every 10-20 years. Economic issues are still what is pressuring
farmers. In terms of irrigation, pumping costs are a major driver. Variable rate pumps should
be used to take advantage of low rate, low energy options.
Along with Bob Farved from the Biofuels and Renewable Feedstocks program, we discussed
the development of this industry in the US and the ways in which it interacts with the water
sector. At this stage there is an aim to develop dryland crops to reduce dependence and
impact of biofuels on water resources. Along with new crops, sorghum is being considered
as it is relatively drought tolerant. There is no research being undertaken to support corn
based oils, and they are sticking to native species as much as possible. There is pressure to
work on species such as Miscanthus (Giant Reed) – but the invasive nature of this crop is a
risk, and the research is trying to minimise this risk (especially due to the fact that research
is funded by the public sector). The limitation for biofuel production is ultimately water, and
there is concern about the biofuel-energy-water nexus – they are trying to build models to
get an idea of capacities and limitations. There are a number of production options to meet
biofuel production needs, and one option is to help farmers identify ways of developing local
fuel options on/near farm systems.
Professor David Pimentel from Cornell University also discussed biofuel use in the US. He is
openly opposed to current biofuel policies – US$23 billion subsidies for corn ethanol, and
soybean biodiesel. This has increased food costs by around US$9 billion in addition to the
subsidies (mainly through higher meat, milk and egg prices). His research indicates that
biofuel production is energy negative (i.e. more energy is required in the process than is
produced by the biofuels). Possibly the only energy "positive" crop for biofuels is algae.
Analysis shows that the cost of production ranges from $0.2 - $9/l – their analysis suggests
$1.8 which could make it feasible. Professor Pimentel would like to see diesel prices at
around $10/l to encourage sustainable use of diesel fuel and also to make alternatives more
6.2.4 Water tensions in California
Mike Wade from the California Farm Water Coalition (FWC) explained some of the
pressures being experienced by irrigators in California, and also arranged several field visits
for me. The FWC was formed in 1989 for education and public relations purposes, to try and
address the negative publicity over agricultural water management. It is funded by dues
(40c/ha) from irrigation councils. Mr Wade also works as part of the Agricultural Water
Management Council (established in 1998), which works on water use efficiency projects,
water management planning and deals at the district water supply level.
Water rights in California are a right to use, not a property right. The right to ‘beneficial' use
is defined by the state water code, and include appropriative, riparian and contract rights.
The biggest problem facing irrigators currently are changing environmental regulations,
including quality and environmental flows. There is not a water supply issue; there is a water
legislation problem. Due to what are seen as environmental issues in the Delta region of the
state, water allocations for irrigation have been cut to just 10% even in average climatic
years. Agriculture is being blamed for decline in environmental sustainability, and pumps are
turned off. This is affecting the west side of the San Joaquin Valley, with around 1 million
acres affected. As an example, I visited the town of Firebaugh, who are facing huge social
problems due to massive areas of land being retired because of a lack of water availability –
up to 30,000 acres has been taken out or irrigated production in the area. There is a need to
put plans into place so that actions can be taken in such situations.
An interesting point in terms of PR for the irrigation sector is defined as the ‘hierarchy of
credibility'. This essentially means that there are three levels of motivation for action – the
highest level being the most credible to the general public:
1. The "right" thing to do – environmentalists have capitalised on this
2. Good for my bottom line – what farmers are saying
3. The law says I have to do it – least credible
Environmentalists are operating on a higher level of credibility than farmers, even though
farmers are often doing things for the ‘right' reasons as well. It is essential for farmers to
promote this message.
At the Wheeler Ridge-Maricopa Water Storage District, Bill Taube (General Manager) gave
me some insights into some of the issues he faces along with other water district managers
in the southern San Joaquin Valley. The supply issues due to environmental regulations are
a serious issue in the region; farmers (and the supply company) pay for their full allocation of
water whether it is delivered or not. Water costs for farmers range from $140 - $300 per acre
feet ($113 - $243/ML). The range is due to geographical area, different bond areas and
pumping lift. The challenges for the water supply company are to supply alternative water
sources for their customers in a time of shortage (source water from federal water scheme,
state water scheme, groundwater, aquifer storage and recovery system). Despite massive
over-extraction of groundwater, Mr Taube believed that groundwater regulation was a bad
idea, as each basin is unique and regulation tends to be one size fits all. He thinks there
should be adjudication, not regulation. As an example of the tensions over water in
California, this agency (in conjunction with others) is currently involved in 26 lawsuits.
Figure 14 The California Aqueduct.
6.2.5 Field visits
Mike Wade from the California Farm Water Coalition organised field visits to several
irrigators in the Fresno region, as well as to the Wheeler Ridge-Maricopa Water Storage
District in the southern San Joaquin Valley.
Stamoules Produce is a family run irrigation business. The farm area is 15,200 acres (6151
ha); some of this is double cropped per year, so annually they are irrigating 17,900 acres
(7243 ha) in total each year. Crops produced include sweetcorn, peppers, melon and
broccoli. Total water use is 37,500 acre feet per year (46,255 ML); water is at a premium in
terms of cost and availability. Annual surface water allocation is 9,750 acre feet (12,026 ML).
The balance is pumped or bought (in California there is no restriction on groundwater
pumping). Water costs over $400/acre feet ($325/ML), making up 15% of total cost. Major
energy costs are for cooling (pack house) and pumping (electricity costs for pumping are
$160/acre foot ($130/ML)). The major expenses are labour, water and fertiliser.
All crops are pre-irrigated with sprinklers but then drip is used; this has been in operation for
the past twelve years. The aim of this is to save water (30-40%) and improve production in
terms of yield and quality. Economically, drip is sensible; if $1,000/acre is invested in drip, it
is returned in one year. The company has rented some land with furrow, but if this is rented
for more than 3 years they will convert to drip. For the high value crops produced, drip is
relatively inexpensive. Irrigation is used as a vehicle to transport elements for plant growth –
it is more than just getting the soil wet. The company sees drip as a good fit for their
business. They have made it work, but have worked hard at doing that – to use drip
successfully, they identify a need to have the right attitude, and drip has to fit the crop.
This company is a major operation and is using very advanced practices. An interesting
technique being used is airjection – injecting air into buried driplines (under research at
CSU-F). This technique is reported to give higher yields (30%), 50% more root mass, and
maintain yields with poor quality water, and appears to be a simple riser inserted into the
sub-main that adds air to the system (Figure 15).
Figure 15 "Airjection" at Stamoules Produce.
South of Fresno, I visited Errotabere Ranches. This farm has implemented drip on all land
over the last 3 years. Before this they were using sprinkler and flood, but these systems
were too inefficient. Energy costs were a consideration in the conversion. Water is delivered
pressurised but they add extra pressure with booster pumps. Speed, labour management
and efficiency/distribution uniformity has made drip a good proposition. Major crops are
almonds, pistachios, pomegranates, garlic, tomatoes, garbanzo beans, cantaloupes and
lettuce. Yields have increased with the use of drip, for example garlic yields have increased
from 8 to 12.25 tonnes/acre using half the previous water use. Initially it was planned to use
1 year drip, but they are now moving towards 5-7 years.
Figure 16 Drip irrigation installed at Errotabere Ranch.
7 Lessons learnt & opportunities for Australia
One of the key findings from this travel opportunity was the chance to explore where
different countries are placed in terms of
1. Considering energy and emissions in terms of irrigation, and
2. Assessing these aspects.
While each country and institution differs in its approach, it was revealed that there is a
definite need to establish a better understanding of the energy and emission intensities of
different crop production systems and the effects of irrigation method/water source on these
intensities. This is particularly true given the pressures on fossil fuel resources, biofuel
development and emission mitigation strategies under consideration. It is also confirmed that
given the wide range of crop production systems found globally, and the different
approaches taken to their management, the issue of up scaling variable local results is vital.
This was discussed with several of the scientists I met, and the recommended options for
incorporating variability into regional/global scales are:
To be extremely careful to be specific as to which crop and region that is being
discussed, including the irrigation technology being used.
To ensure greater accuracy, more information must be known about a region when
there is high variability. It is essential to know the system well in general, as well as
to have comprehensive direct measurements on farm.
Looking at the use of different irrigation methods across the globe gives some options for
adapting irrigation to a low energy future. For example, the use of low pressure drip systems
can offer an opportunity to reduce water and energy use. There is also evidence to suggest
that emissions are reduced with the use of drip irrigation (Kallenbach et al., 2010). However,
these systems clearly still have some limitations, for example the need for good quality
water, and like any irrigation method, obviously require high standards of management to
make them successful. It is important to recognise that there is no one size fits all approach.
Aside from pumping, nitrogen fertiliser use is a major component of energy consumption,
and is also associated with nitrous oxide emissions. Closely matched nitrogen (and other)
fertiliser application to plant nutritional requirements can optimise the use of nitrogen,
ensuring that maximum benefit is derived from its application. This also prevents pollution of
groundwater and surface water from leaching of fertilisers that are applied in the wrong
quantities and at the wrong times.
The experience of the California Agricultural Pumping Efficiency Program shows that it is
vital to look at all system components when considering irrigation. By ensuring that pumps,
motors and irrigation systems are properly designed, installed and maintained, there are
opportunities for water and energy savings. In California alone, this program has saved over
1 million kWh per month from the electric pumps that have been tested to date. Assessment
of irrigation pumps in some parts of Australia has shown that up to 50% are performing
poorly. A similar program to that developed in California, if implemented in Australia, could
deliver electricity and diesel savings, reducing pumping costs for irrigators and reducing
associated emissions.
Other key learnings included;
The differences in scale between the countries visited. For example, Israel has just
182,000 ha of irrigated land, compared to 22.4 million ha in the USA. Along with total
country size and resources, and governance/legislation differences, this question of
scale raises questions about the feasibility of managing irrigation in such a high-tech
was as Israel, on a bigger scale.
The differences in the way water rights/legislation is structured was also an eye
opener; unlike Australia, the countries visited had the right to use water for irrigation,
but did not actually own the right as irrigators do here. In Israel, the government
"owns" all water, including surface and groundwater. In California, there is no
restriction on the use of groundwater. This has huge implications for over extraction,
and there are problems with land subsidence and degraded water quality as a result.
Despite this, groundwater regulation in terms of legislation was seen by some as a
threat due to the different requirements for each region; legislation is seen as a "one
size fits all approach" which would not account for this variability.
The economic impact of water use – for example, in the UK, while irrigation is a very
small portion of water use (2% of total), the difference in price between irrigated and
non-irrigated strawberries is up to £3,000/ton. Thus the security of access to water at
critical times is vital for these farmers.
The current global focus on water and food shortages in conjunction with rising energy costs
and a drive to reduce emissions means that there is a requirement to understand these
impacts on the irrigation sector. Strategic research in this area will be necessary in order to
achieve a balance between meeting economic, social, environmental and legal obligations.
A better understanding of these challenges will help with the development of strategies to
adapt to changes brought about by a changing climate and related policies. This fellowship
allowed me to interact with others around the world who are working on aspects of the
energy-water nexus. Ultimately, it would be beneficial if these interactions and discussions
could lead to options for the continued development of sustainable, vibrant agricultural
communities through knowledge sharing and new science. Policies and practice for the
irrigation industry must promote the production of food and fibre according to best-practice
management with minimum impact to the environment.
8 Bibliography
Amaya C (2000) 'A Life Cycle Analysis of Energy Use by Irrigation Systems.' Department of
Civil and Environmental Engineering, University of Melbourne, Melbourne, Victoria.
Canakci, M., Topakci, M., Akinci, I., & Ozmerzi, A. (2005). Energy Use Pattern of Some Field
Crops and Vegetable Production: Case Study for Antalya Region, Turkey. Energy
Conversion and Management, 46(4), 655-666.
Center for Irrigation Technology (No date). Agricultural Pumping Efficiency Program. Center
for Irrigation Technology, California State University, Fresno.
Chamsing A, Salokhe V, Singh G (2006) Energy Consumption Analysis for Selected Crops
in Different Regions of Thailand. Agricultural Engineering International: the CIGR Ejournal 8.
Chaudhary V, Gangwar B, Pandey D (2006) Auditing of Energy Use and Output of Different
Cropping Systems in India. Agricultural Engineering International: the CIGR Ejournal 8.
Chen G, Baillie C (2007) 'Development of EnergyCalc - A tool to assess cotton on-farm
energy uses.' University of Southern Queensland, Toowoomba.
Chen G, Kupke P, Baillie C (2008) 'Opportunities to Enhance Energy Efficiency and
Minimise Greenhouse Gases in Queensland's Intensive Agricultural Sector.' National Centre
for Engineering in Agriculture, University of Southern Queensland, Toowoomba.
Conforti P, Giampetrio M (1997) Fossil energy use in agriculture: an international
comparison. Agriculture, Ecosystems and Environment 65, 231-243.
CSIRO (2007) 'Climate Change in Australia.' CSIRO, Canberra.
DEFRA (2009). Sustainable Farming and Food Strategy – indicator data sheet
Department of the Environment, Water, Heritage and the Arts (2008) Retrieved 17/11/2008,
Entry JA, Sojka RE, Shewmaker GE (2002) Management of irrigated agriculture to increase
organic carbon storage in soils. Soil Science Society of America Journal 66, 1957-1964.
Erdal, G., Esungun, K., Erdal, H., & DGunduz, O. (2007). Energy use and economical
analysis of sugar beet production in Tokat province of Turkey. Energy, 32, 35-41.
Esungun, K., Erdal, G., Gunduz, O., & Erdal, H. (2007). An economic analysis and energy
use in stake-tomato production in Tokat province of Turkey. Renewable Energy, 32, 1873-
General Directorate of State Hydraulic Works, Turkey (2009a), Turkey Water Report 2009,
General Directorate of State Hydraulic Works, Turkey.
General Directorate of State Hydraulic Works, Turkey (2009b), Water and DSI, General
Directorate of State Hydraulic Works, Turkey.
Hamdy A, Ragab R, Scarascia-Mugnozza E (2003) Coping with water scarcity: Water saving
and increasing water productivity. Irrigation and Drainage 52, 3-20.
Hatirli, S. A., Ozkan, B., & Fert, C. (2006). Energy inputs and crop yield relationship in
greenhouse tomato production. Renewable Energy, 31, 427-438.
Hellegers P, Zilberman D, Steduto P, McCornick P (2008) Interactions between water,
energy, food and environment: evolving perspectives and policy issues. Water Policy 10, 1-
Hess, T.M., Knox, J.W., Kay, M.G. and Weatherhead, E.K. (2009). Managing Water Better.
The agronomic, economic and environmental benefits of good irrigation scheduling. An
information booklet produced for DEFRA Innovation and the Environment Agency. Cranfield
Hodges AW, Lynne GD, Rahmani M, Casey CF (1994) Adoption of Energy and Water-
Conserving Irrigation Technologies in Florida. University of Florida
s+Agriculture+in+the+21st.htm [Accessed 19/06/2010]
Jackson TM, Khan S, Hafeez M (2010) A comparative analysis of water application and
energy consumption at the irrigated field level. Agricultural Water Management.
Kallenbach, C.M., Rolston, D.E., & Horwath, W.R. (2010). Cover cropping affects soil N2O
and CO2 emissions differently depending on type of irrigation. Agriculture, Ecosystems &
Environment, 137(3-4), 251-260.
Khan S, Khan MA, Hanjra MA, Mu J (2009) Pathways to reduce the environmental footprints
of water and energy inputs in food production. Food Policy 34, 141-149.
Lal R (2004) Carbon emission from farm operations. Environment International 30, 981-990.
Martin JF, Diemont SAW, Powell E, Stanton M, Levy-Tacher S (2006) Emergy Evaluation of
the Performance and Sustainability of Three Agricultural Systems With Different Scales and
Management. Agriculture Ecosystems & Environment 115, 128-140.
Michael Young and Associates (2005) A Discussion on the Energy and Green House Gas
Effects Arising From the Adoption of New Irrigation Technology by the Dairy Farming
Industry. Department of Primary Industries, Victoria, Tatura.
Naylor RL (1996) Energy and resource constraints on intensive agricultural production.
Annual Review of Energy and the Environment 21, 99-123.
Oenema O, Velthof G, Kuikman P (2001) Technical and policy aspects of strategies to
decrease greenhouse gas emissions from agrciculture. Nutrient Cycling in Agroecosystems
60, 301-315.
Ozkan, B., Akcaoz, H., & Fert, C. (2004). Energy input-output analysis in Turkish agriculture.
Renewable Energy, 29, 39-51.
Ozkan, B., Fert, C., & Karadeniz, C. F. (2007). Energy and cost analysis for greenhouse and
open-field grape production. Energy, 32, 1500-1504.
Phocaides A (2001) 'Handbook on pressurized irrigation techniques.' (Food and Agriculture
Organisation of the United Nations: Rome)
Pimentel D (1992) Energy inputs in production agriculture. In 'Energy in Farm Production'.
(Ed. RC Fluck). (Elsevier: Amsterdam)
Pimentel D, Doughty R, Carothers C, Lamberson S, Bora N, Lee K, Hansen D, Uphoff N,
Slack S (2002) Energy Inputs in Crop Production in Developing and Developed Countries. In
'Food Security and Environmental Quality in the Developing World'. (Ed. R Lal) pp. 129-151.
(CRC Press: USA)
Pimentel D, Hepperly P, Hanson J, Douds D, Seidel R (2005) Environmental, Energetic, and
Economic Comparisons of Organic and Conventional Farming Systems. Bioscience 55, 573-
PImentel D, Houser J (1997) Water Resources: Agriculture, The Environment, and Society.
Bioscience 47.
Pimentel D, Hurd LE, Bellotti AC, Forster MJ, Oka IN, Sholes OD, Whitman RJ (1973) Food
Production and the Energy Crisis. Science 182, 443-449.
Pimentel D, Marklein A, Toth MA, Karpoff MN, Paul GS, McCormack R, Kyriazis J, Krueger
T (2009) Food versus Biofuels: Environmental and Economic Costs. Human Ecology 37, 1-
Pimentel D, Pimentel M (2008) 'Food, Energy and Society.' (CRC Press: Boca Raton, FL)
Pratt Water (2004) 'The Business of Saving Water: The Report of the Murrumbidgee Valley
Water Efficiency Feasibility Project.' Pratt Water, Campbellfield, Victoria.
Sayin, C., Mencet, M. N., & Ozkan, B. (2005). Assessing of energy policies based on Turkish
agriculture: current status and some implications. Energy Policy, 33, 2361-2373.
Singh H, Mishra D, Nahar NM (2002) Energy use pattern in production agriculture of a
typical village in arid zone, India - part I. Energy Conversion and Management 43, 2275-
Singh H, Singh AK, Kushwaha HL, Singh A (2007) Energy consumption pattern of wheat
production in India. Energy 32, 1848-1854.
Smith, P., Martino, D., Cai, Z., Gwary, D., Janzen, H., Kumar, P., et al. (2007). Agriculture in
Climate Change 2007: Mitigation. Contribution of Working Group III to the Fourth
Assessment Report of the Intergovernmental Panel on Climate Change. Cambridge, United
Kingdom and New York, NY, USA.
Srivastava RC, Verma HC, Mohanty S, Pattnaik SK (2003) Investment decision model for
drip irrigation. Irrigation Science 22, 79-85.
Tzilivakis J, Warner DJ, May M, Lewis KA, Jaggard K (2005) An assessment of the energy
inputs and greenhouse gas emissions in sugar beet (Beta vulgaris) production in the UK.
Agricultural Systems 85, 101-119.
USDA (2009) 2007 Census of Agriculture 2008 Farm and Ranch Irrigation Survey (2008)
[Accessed 05/07/2010]
USDA (2009) 2007 Census of Agriculture 2008 Farm and Ranch Irrigation Survey (2008)
tion_Survey/fris08.pdf [Accessed 05/07/2010]
Williams, A.G., Pell, E., Webb, J., Tribe, E., Evans, D., Moorhouse, E., and Watkiss, P.
(2008). Final Report for Defra Project FO0103, Comparative Life Cycle Assessment of Food
Commodities Procured for UK Consumption through a Diversity of Supply Chains. London,
Zehnder AJB, Yang H, Schertenlieb R (2003) Water issues: the need for action at different
levels. Aquatic Sciences 65, 1-20.
Source: http://irrigation.org.au/documents/publications-resources/conference_papers_2010/100610_PlenarySession4_TravelFellowshipReport_TamaraJackson.pdf
Journal of Animal Ecology 2010, 79, 82–87 Differential effects of moving versus stationary territorialintruders on territory defence in a songbird Valentin Amrhein* and Sabine Lerch Research Station Petite Camargue Alsacienne, Rue de la Pisciculture, 68300 Saint-Louis, France, and University of Basel,Zoological Institute, Vesalgasse 1, 4051 Basel, Switzerland 1. In territorial contests, not only acoustic or other signals, but also the movements of a territorialintruder are likely to influence the response of a resident.2. We tested this movement hypothesis by simulating moving vs. stationary intruders into the ter-ritories of winter wrens Troglodytes troglodytes, using the same non-interactive song playbacks inboth treatments.3. Male winter wrens showed a different long-term singing reaction in response to a moving thanto a stationary intruder.4. One day after experiencing an intruder that was switching between three locations, residentsstarted to sing earlier before sunrise, and they sang more and longer songs at dawn than before theintrusion.5. Residents receiving the same playback from one location only reacted by starting to sing laterrelative to sunrise, and by singing fewer and shorter songs than before the intrusion.6. We could not discriminate between the treatments when examining the short-term singing reac-tions during and immediately after the playbacks. However, our results clearly demonstrate aneffect of the spatial behaviour of territorial intruders on the long-term territory defence of residentsat dawn, about 24 h after an intrusion.7. We argue that spatial behaviour of territorial intruders should be an integral part of the studyof animal territory defence behaviour. Investigating long-term changes in territory defence at dawnis a sensitive tool for discriminating between different types of intruders.
GREEN MEDICINE: USING LESSONS FROM TORT LAW AND ENVIRONMENTAL LAW TO HOLD PHARMACEUTICAL MANUFACTURERS AND AUTHORIZED DISTRIBUTORS LIABLE FOR INJURIES CAUSED BY COUNTERFEIT DRUGS Stephanie Feldman Aleong* The majority of the American public would be astonished by the frequency with which counterfeit prescription drugs appear on reputable drugstore shelves. In 2004, the Food and Drug Administration (FDA) noted thatthose who counterfeit prescription drugs "deny ill patients the therapies thatcan alleviate suffering and save lives."1 In 2006, the World HealthOrganization (WHO) estimated that there exists a $30 billion market in fakedrugs.2 Although the FDA has tried to characterize the incidence of