Doi:10.1016/j.tim.2005.10.00
TRENDS in Microbiology
Vol.13 No.12 December 2005
Towards a comprehensive viewof the bacterial cell wall
Boris Dmitriev1, Filip Toukach2 and Stefan Ehlers3
1N.F. Gamaleya Institute for Epidemiology and Microbiology, Gamaleya str. 18, Moscow 123098, Russia2N.D. Zelinsky Institute for Organic Chemistry, Leninsky Prosp. 33, Moscow 119991, Russia3Division of Molecular Infection Biology, Research Center Borstel, Parkallee 22, D-23845 Borstel, Germany
Direct in vivo visualization, in full atomic detail, of the
different concept for the architecture of bacterial cell
microbial cell wall and its stress-bearing structural
walls, known as the scaffold model As a result of re-
architecture remains one of the prime challenges in
evaluating experimental evidence, this model shows
microbiology. In the meantime, molecular modeling can
glycan chains within murein of either Gram-negative or
provide a framework for explaining and predicting
Gram-positive bacteria running perpendicular to the
mechanisms involved in morphogenesis, bacterial cell
plasma membrane. Cross-linked by peptide bridges, they
growth and cell division, during which the wall and its
produce a continuous sponge-like matrix (not layers) that
major structural component – murein – have to protect
can function as an elastic external cytoskeleton.
the cell from osmotic pressure and multiple tensile
Obviously, the traditional and novel models are
forces. Here, we illustrate why the scaffold concept of
mutually exclusive. However, because the chemical
murein architecture provides a more comprehensive
parameters of the two models are closely related, and
representation of bacterial cell wall physiology than
because there is no direct experimental approach to
previous models.
distinguish between them, a stimulating, if controversial,discussion of the two models has been initiated in theliterature In our opinion, it is time to put themodels to use for explaining and predicting basic facets of
bacterial cell physiology. Here, we show how the scaffold
The cell wall of bacteria represents a structural unity of
model fulfills this demand in the paradigmatic case of
variable thickness, which is located outside the plasma
Gram-negative Escherichia coli.
membrane and completely covers the cell. It is both firm-to-rupture and elastic, thereby preventing the cell fromdisintegration by the intracellular osmotic pressure .
The major stress-bearing component of the bacterial
Murein determines the shape of the bacterial cell.
cell wall is murein, a chemical synonym of which is
Although intuitively evident for symmetrical cells that
peptidoglycan (PG). In 1964, Weidel and Pelzer
possess a classical (round or rod-like) morphology, this
proposed that PG strands within the ‘bag-shaped' murein
statement must also be true for the branched type of
(sacculus) run parallel to the plasma membrane. Being
morphology, when cells exhibit Y-, X- or H-like shapes
cross-linked by peptide bridges, glycan chains were
caused by mutations of specific genes encoding the
thought to make thin networks (layers). Glycan chains
synthesis of murein-assembling enzymes. The morpho-
were assumed to run perpendicular to the long axis of the
logical transformations observed for the rod-like E. coli
cell, whereas peptide bridges were arranged in parallel
cells are particularly impressive: after a set of
The major obstacle for direct experimental proof of
mutations they grew either as filaments or branched
this postulated structure is that a living cell does not
dendrites or large spheres, the spheres dividing like
possess a fixed cell-wall structure because the cell wall is
Neisseria or Staphylococcus (i.e. with a successive
in a state of permanent biosynthesis, assembly, disas-
alteration of the division plane orientation). These radical
sembly and turnover. This makes the cell-wall architec-
morphological transformations are solely possible if the
ture of each individual cell within a population both
stress-bearing murein possesses a kind of universal
heterogeneous and irregular.
tertiary structure, which effortlessly tolerates and enables
During the past four decades, several experimental
major morphological perturbations. The major structural
observations have accumulated that tend to contradict the
principle of murein architecture has to be universal and
adopted structural paradigm of PG layers (reviewed in
valid for all types of bacterial cell morphology.
). It is, therefore, timely and necessary to readdress thecrucial question of how the murein tertiary structure
General chemical and physical principles of murein
copes in vivo with the major processes and tensile forces of
bacterial cell physiology. Recently, we proposed a radically
Although the primary chemical structure of murein mightvary in different organisms, the material, regardless of the
Corresponding author: Ehlers, S. (
[email protected]).
Available online 19 October 2005
taxonomy and morphology of a given bacterial cell,
0966-842X/$ - see front matter Q 2005 Elsevier Ltd. All rights reserved. doi:10.1016/j.tim.2005.10.001
TRENDS in Microbiology
Vol.13 No.12 December 2005
The predominant occurrence of short chains translates
Box 1. Characteristic structural elements of murein
into numerous cuttings within the network. The number
(i) A peptidoglycan (PG) strand is both a regular and symmetrical
of large holes increases dramatically when the degree of
molecule. Every strand consists of alternating disaccharide-peptide
murein cross-linking is reduced to the levels observed in
units; disaccharide fragments are connected into regular glycan
nature, and in this case the network becomes dysfunc-
chains, and peptide substituents project outward from each oddmonosaccharide residue The disaccharide-repeating unit
consists of N-acetylmuramic acid (blue disk, beginning of the
The situation is radically different in the case of the
chain) and N-acetylglucosamine (red disk).
scaffold model, which does not crucially depend on the
(ii) According to X-ray diffraction data, conformation of the strand
strand lengths and readily accommodates both the short
represents a right-handed helix with the symmetry order C4, each
chains and a lower degree of cross-linking (The
turn of the helix consisting of four disaccharide units with fourpeptide side-chains oriented outward A strand with two
crucial questions are: (i) how are the glycan strands
turns of the helix is shown in the insert.
oriented relative to the plasma membrane and each other
(iii) Strands are of different lengths: oligomers comprising 8–12
and (ii) what is the murein architecture like?
disaccharide units predominate but short chains and longpolymers are also present.
Structural paradigm for murein architecture in rod-like
(iv) The formation of peptide bridges is readily possible because
each peptide arm possesses both free amino (filled circles) and
Gram-negative bacteria
carboxyl (empty circles) groups.
The murein of all Gram-negative bacteria belongs to the
(v) Not all adjacent peptides are bridged, therefore, the degree of
simplest chemical type The cell wall of E. coli has
cross-linking is variable.
been studied comprehensively by electron microscopy and
(vi) Some crucial physical parameters are as follows: the length
and the width of one disaccharide unit is 1.0 and 1.1 nm, respectively
biochemical methods. The actual distance between the
the lengths of the peptide arm and the expanded bridge are 2.2
inner and outer membranes (i.e. the periplasm height in
and 4.36 nm, respectively
hydrated E. coli cells deeply frozen at ambient pressure)was reproducibly measured as 33 nm . Furthermore,the observed width of the major murein body was 8 nm,and the material was located close to the outer membrane.
Here, it was centered around the ends of the peptidemoieties of lipoprotein molecules, whose protein core is8 nm The bulk of murein extended toward the plasmamembrane and gradually became less dense; the overallthickness of the whole murein mass was w18 nm Evidently, this material is a major structural component ofthe periplasm and represents the resilient ‘periplasmicgel' of the bacterial envelope
Combining the principles of the scaffold-like murein
architecture and the experimentally determined par-ameters of the E. coli periplasm detailed previously, wenow present the first graphical in-scale depiction of theGram-negative envelope (Traditional cartoonsof the Gram-negative envelope depict the periplasmic
TRENDS in Microbiology
space as essentially empty with a thin murein layer insidetherefore, our presentation in is
Figure I. General view of a separate peptidoglycan (PG) strand.
radically different from all previous models. however, readily illustrates that the periplasmic space isprone to compression. It is therefore easy to understand
invariably comprises PG strands cross-linked by peptide
that, when E. coli cells were rapidly frozen at a high
bridges The chemical features of PG strands are
pressure, the height of the periplasm dropped to 20 nm
and the visible zone of murein was reduced to 6 nm .
The murein sacculus is a cocoon-like construction,
The murein architecture resembles a sponge-like
which has to contain and oppose the rupturing forces,
matrix, the height of the matrix being proportional to
therefore, its architecture must correspond to the mech-
the glycan-chain lengths. How can the scaffold-like
anical principles of safe engineering and constructing.
murein architecture be assembled during continuous
Therefore, stress-bearing elements within murein of the
bacterial cell growth and division? There are two
rod-like bacterium must be arranged differently than
peculiarities that compound this problem: (i) the murein-
suggested by the classical model and, if we remain within
assembling enzymes are membrane-bound proteins that
the confines of this model, these elements should adopt a
use precursors from the cytoplasm, and (ii) the wall, which
hexagonal architecture (Analogous consider-
is being assembled, is located in the periplasm at a
ations have led Koch to propose the term ‘chicken-wire'
substantial distance from the membrane. Before answer-
network It is clear that the stress-bearing properties
ing the question, we would like readers to recall that
of the ‘chicken-wire' architecture directly depend on the
cylindrical and pole regions of the rod-like cell are
lengths of PG-strands. These, however, have been
synthesized by two distinct mechanisms: (i) patch-
demonstrated experimentally to be rather short .
insertion mode of growth and (ii) zonal mode of growth
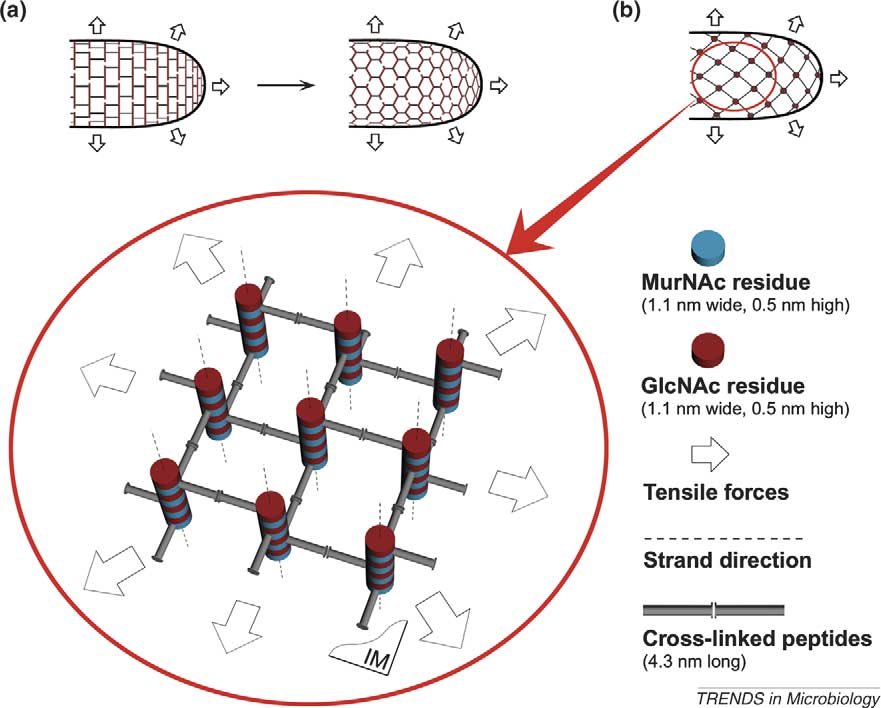
TRENDS in Microbiology
Vol.13 No.12 December 2005
Figure 1. Arrangement of the stress-bearing elements within the cell wall of a rod-like bacterium. (a) Glycan chains run as postulated by the classical model, that is, parallel tothe plasma membrane and the short axis of the cell (not along the tensile forces). Transformation to the ‘chicken-wire' architecture is most probable. (b) Glycan chains arearranged perpendicular to the plasma membrane, peptide bridges being the stress-bearing elements of the construction (scaffold model). The orientation of bridges is inaccord with the direction of tensile forces. A small fragment of the murein architecture is enlarged in the circle. Nine cross-linked peptidoglycan (PG) strands are clearly seen,plasma membrane (IM) being underneath the strands. For the purpose of clarity, only one turn of each strand is depicted, otherwise numerous crossed lines obscure thepicture. In fact, strands are longer and the number of bridges is bigger. Four helical pores (channels) are seen round the central strand.
via attachment of the nascent strands to the leading edge
patchiness of murein en masse insertions into the
sidewall of E. coli
To explain the mode of cylindrical growth, the concept
Regarding a secure mechanism for cell-wall division,
of membrane-adhesion zones developed by Bayer is
we propose that, after chromosome segregation, intensive
appropriate . According to this concept, the inner
synthesis of murein is triggered, culminating in septum
membrane (IM) is able to bulge and approach the outer
formation. During the constriction of the cell, the leading
membrane (OM), effectively making a kind of OM–
edge of the murein structure and the curved bend of
murein–IM multi-enzyme complex The initial local
the plasma membrane are clearly exposed, both of them
hollow space within the murein can be produced, for
adopting the form of concentric rings. The septum grows
example, by the soluble transglycosylase Slt70 and
strictly centripetally, like the iris diaphragm of a camera,
endopeptidase; both enzymes are known to participate
from the peripheral edges of the murein to the center
in gradual murein degradation . The cavity is
In the case of septum formation, the murein-synthesizing
expected to expand by the turgor pressure, thus
complexes, such as the members of FtsZ-ring and
enabling the inner membrane to bulge. If the murein-
associated counterparts , are probably located not at
the top of membrane bulges (as is the case for cylindrical
protrusion, they can simultaneously use precursors
growth) but at the invaginated membrane curve.
coming from the cytoplasm and be in contact with the
The proposed concept of bacterial cell-wall morphogen-
pre-existing murein, which functions as the acceptor for
esis is in agreement with well-documented observations
the nascent strands. As the newly synthesized murein
that the process of a gradual E. coli cells lysis is paralleled
starts to fill the expanded cavity, the convex membrane
by the release of simple muropeptides and peptides with
gradually returns to its original position. As soon as the
concomitant increase in the cross-linking index of the
perforated murein is mended and a large piece of new
remaining cell walls Moreover, in the course of lysis,
material is inserted, the current round of murein growth
walls become progressively thinner from inside to outside
is completed and the inner membrane bulges appear
whereas no visible holes and long cuts were observed
in other places to repeat new cycles. Metaphorically
on the isolated sacculi . It is clear from that
speaking, the membrane loaded with the murein-
lytic degradation of the murein from inside by Slt70 and
synthesizing complexes functions as a sewing machine,
endopeptidase will result in the release of simple
new murein being assembled in a direction from the OM
degradation products, and the surviving walls become
to the IM To the best of our knowledge, this
thinner but relatively more cross-linked in comparison to
is the only mechanism that explains the random
the original murein. It is difficult to explain these
TRENDS in Microbiology
Vol.13 No.12 December 2005
Highly cross-linked zone
Murein precursors
Newly synthesized
TRENDS in Microbiology
Figure 2. Proposed molecular architecture of a Gram-negative envelope and a tentative mechanism of bacterial cell wall growth. (a) The asymmetric outer membrane (OM)consists of two leaflets, the external one comprising lipopolysaccharide molecules (blue) and the internal leaflet comprising phospholipids (green). The essential componentof the internal leaflet is lipoprotein (brown), the peptide moiety of which protrudes into the periplasm. Different transmembrane porins, either trimeric or monomeric, arecommon components of the OM. The inner membrane (IM, green) comprises phospholipids and is penetrated by different transmembrane proteins, of which two permeasesare presented (black serpentine). Permeases are thought to locate strictly beneath the corresponding porins. The major component of the periplasm is the hydrated‘periplasmic gel'(blue diffuse zone) consisting of murein, the bridged PG strands of which run perpendicular to the IM. Certain central bridges are covalently connected to theends of lipoprotein molecules that protrude into the periplasm. The height of the periplasm is variable and depends on external conditions. (b) Dynamics of the lateral mureingrowth by the patch-insertion mechanism is clearly seen from two ‘snap-shot' pictures. On the left, the plasma membrane locally bulges to fill the cavity produced within theold cell-wall by a lytic enzyme bound to the periplasm. The existing turgor pressure is a driving force for both the cavity expansion and membrane bulging. The murein-synthesizing complexes (MSC ovals) locate on the bulge top, therefore having the possibility of translocating the biosynthetic murein precursors from the cytoplasm acrossthe membrane with concomitant synthesis of the novel PG strands and their immediate attachment to the old wall edges. On the right, the novel murein patch is growing byelongation of the inserted strands, thus filling the cavity and pushing the membrane bulge downward until the plasma membrane returns to its original state.
TRENDS in Microbiology
Vol.13 No.12 December 2005
Table 1. Experimental observations and murein models: compatibility
Observation and properties
Arrangement of the strands and peptide bridges
Conflict (classical), Agreement (chicken-wire)
along tensile forcesBranched-cell morphology
Successive alteration of the division plane
orientationRelease of 100-kDa proteins by hypo-osmotic
shockIn silico modeling of murein assembly from
separate strands to form stress-bearing matrixReduction by 50% of murein content per unit of
observations from the position of the classical model
gradients being built up within the murein. The existence
because it implies that the cell wall is already thin and
of both the compartmentalization and the gradient of
that lytic enzymes cut it along the glycan strands, much
electrical potential is a prerequisite for the effective influx
like scissors cut paper.
of necessary nutrients across the periplasm; this is alsotrue for the trafficking of polymers via distinct secretionpathways. These features of the proposed murein archi-
Current status of the scaffold model
tecture offer new links to further biophysical and
We have also tentatively simulated the layered murein
biochemical studies of the functions of bacterial cytoplasm
architecture according to the traditional network-model,
and envelope, particularly those high-resolution technol-
and a comparison of the two models is presented in
ogies that are aimed at unravelling the problem of how
. Although the scaffold concept seems to have clear
cells are able to control precisely both the predetermined
advantages over the traditional model, we do not wish to
form and the constancy in length and width of their
imply that it is unconditionally superior. Repeated
experimental feedback and modeling input from cell wallexperts of divergent opinions will be necessary to refine
the model to fully reflect reality.
B.D. and F.T. are recipients of a scholarship from the Research Center
Recently, critics of the model argued that all our
Borstel. S.E. holds a grant from the Deutsche Forschungsgemeinschaft
calculations are based on an average glycan-chain length
of 8–12 disaccharide units, whereas (according to thecritics) this average length is 33 units It is important
to realize, however, that there are two methods for
1 Stanier, R.Y. and van Niel, C.B. (1962) The concept of a bacterium.
determining glycan chain lengths: (i) the direct measure-
Arch. Mikrobiol. 42, 17–35
ment of glycan chains distribution in cell walls digests
2 Weidel, W. and Pelzer, H. (1964) Bagshaped macromolecules – a new
outlook on bacterial cell walls. Adv. Enzymol. Relat. Areas Mol. Biol.
with L-alanine amidase followed by HPLC quantitative
analysis and (ii) an indirect method that determines
3 Ho¨ltje, J-V. (1998) Growth of the stress-bearing and shape-maintain-
the amount of terminal units relative to the amount of all
ing murein sacculus of Escherichia coli. Microbiol. Mol. Biol. Rev. 62,
fragments The first method is rather tedious, but
highly accurate, whereas the second method is simpler but
4 Vollmer, W. and Ho¨ltje, J-V. (2004) The architecture of the murein
(peptidoglycan) in Gram-negative bacteria: vertical scaffold or
prone to overestimation. Evidently, the data obtained by
horizontal layer(s)? J. Bacteriol. 186, 5978–5987
the first method were our prime choice for creating an
5 Dmitriev, B.A. et al. (1999) Layered murein revisited: a fundamentally
accurate model. Several researchers inter-
new concept of bacterial cell wall structure, biogenesis and function.
pret the data in the same way as we do here, namely to
Med. Microbiol. Immunol. (Berl.) 187, 173–181
6 Dmitriev, B.A. et al. (2003) Tertiary structure of bacterial murein: the
imply that the average glycan chain length is in the order
scaffold model. J. Bacteriol. 185, 3458–3468
of 8–12 disaccharides.
7 Dmitriev, B.A. et al. (2000) Molecular mechanics of the mycobacterial
cell wall: from horizontal layers to vertical scaffolds. Int. J. Med.
Microbiol. 290, 251–258
Concluding remarks
8 Dmitriev, B.A. et al. (2004) Tertiary structure of Staphylococcus
The proposed scaffold-like principle of bacterial murein
aureus cell wall murein. J. Bacteriol. 186, 7141–7148
architecture fulfills the requirements of a stress-bearing
9 Matias, V.R. and Beveridge, T.J. (2005) Cryo-electron microscopy
construction, enabling it to be simultaneously porous and
reveals native polymeric cell wall structure in Bacillus subtilis 168and the existence of a periplasmic space. Mol. Microbiol. 56, 240–251
elastic, compact and stretchable. The porous matrix
10 Young, K.D. (2003) Bacterial shape. Mol. Microbiol. 49, 571–580
retains a large amount of water, thus exhibiting the
11 Varma, A. and Young, K.D. (2004) FtsZ collaborates with penicillin
properties of a periplasmic gel, which is able to shrink and
binding proteins to generate bacterial cell shape in Escherichia coli.
swell. The proposed principle is also in agreement with the
J. Bacteriol. 186, 6768–6774
idea of periplasm compartmentalization. The high vis-
12 Schleifer, K.H. and Kandler, O. (1972) Peptidoglycan types of bacterial
cell walls and their taxonomic implications. Bacteriol. Rev. 36,
cosity gel prevents convection and enables only facilitated
diffusion under the guidance of specific substrate-binding
13 Koch, A.L. (1998) Orientation of the peptidoglycan chains in the
proteins and the plasma membrane potential, charge
sacculus of Escherichia coli. Res. Microbiol. 149, 689–701
TRENDS in Microbiology
Vol.13 No.12 December 2005
14 Harz, H. et al. (1990) Isolation and separation of the glycan strands
fraction containing attachment sites between the inner and outer
from murein of Escherichia coli by reversed-phase HPLC. Anal.
membranes and the murein skeleton of the cell envelope. J. Biol.
Biochem. 190, 120–128
Chem. 261, 428–443
15 Obermann, W. and Ho¨ltje, J-V. (1994) Alterations of murein structure
28 Kitano, K. et al. (1986) Transglycosylase and endopeptidase partici-
and penicillin-binding proteins in minicells from Escherichia coli.
pate in the degradation of murein during autolysis of E. coli.
Microbiology 140, 79–87
J. Bacteriol. 167, 759–765
16 Ishidate, K. et al. (1998) Analysis of the length distribution of murein
29 Nanninga, N. (1998) Morphogenesis of Escherichia coli. Microbiol.
glycan strands in ftsZ and ftsI mutants of Escherichia coli. FEMS
Mol. Biol. Rev. 62, 110–129
Microbiol. Lett. 168, 71–75
30 Errington, J. et al. (2003) Cytokines is in bacteria. Microbiol. Mol.
17 Boneca, I.G. et al. (2000) Characterization of Staphylococcus aureus
Biol. Rev. 67, 52–65
cell wall glycan strands, evidence for a new beta-N-acetylglucosami-
31 Leduc, M. et al. (1985) Correlation between degradation and
nidase activity. J. Biol. Chem. 275, 9910–9918
ultrastructure of peptidoglycan during autolysis of Escherichia coli.
18 Dubochet, J. et al. (1983) Electron microscopy of frozen-hydrated
J. Bacteriol. 161, 627–635
bacteria. J. Bacteriol. 155, 381–390
32 Burman, L.G. et al. (1983) Evidence for multisite growth of
19 Shu, W. et al. (2000) Core structure of the outer membrane lipoprotein
Escherichia coli murein involving concomitant endopeptidase and
from Escherichia coli at 1.9A
˚ resolution. J. Mol. Biol. 299, 1101–1112
transpeptidase activities. J. Bacteriol. 156, 386–392
20 Koch, A.L. (2000) Simulation of the conformation of the murein fabric:
33 Glauner, B. (1998) Separation and quantitation of muropeptides with
the oligoglycan, pentamuropeptide, and cross-linked nona-muropep-
high-performance liquid chromatography. Anal. Biochem. 172,
tide. Arch. Microbiol. 174, 429–439
21 Hobot, J.A. et al. (1984) Periplasmic gel: new concept resulting from
34 Pink, D. et al. (2000) On the architecture of the Gram-negative
the reinvestigation of bacterial cell envelope ultrastructure by new
bacterial murein sacculus. J. Bacteriol. 182, 5925–5930
methods. J. Bacteriol. 160, 143–152
35 Burge, R.E. et al. (1977) Structure of the peptidoglycan of bacterial cell
22 Neidhardt, F.C. et al. (1990) Structure and function of bacterial cell
wall. J. Med. Biol 117, 927–953
parts. In Physiology of the Bacterial Cell: A Molecular Approach.
36 Labischinski, H. et al. (1979) On the secondary and tertiary structure
pp. 30–61, Sinauer Associates
of murein. Low and medium X-ray evidence against chitin-based
23 Koronakis, V. et al. (2004) Structure and function of TolC: the bacterial
conformation of bacterial peptidoglycan. Eur. J. Biochem. 95, 147–155
exit duct for protein and drugs. Annu. Rev. Biochem. 73, 467–489
37 Cooper, S. (1997) Division pattern of a round mutant of Escherichia
24 Matias, V.R. et al. (2003) Cryo-transmission electron microscopy of
coli. J. Bacteriol. 179, 5582–5584
frozen-hydrated sections of Escherichia coli and Pseudomonas
38 Demchick, P. and Koch, A. (1996) The permeability of the cell
aeruginosa. J. Bacteriol. 185, 6112–6118
wall fabric of Escherichia coli and Bacillus subtilis. J. Bacteriol.
25 De Pedro, M.A. et al. (2003) Patchiness of murein insertion into the
side wall of Escherichia coli. Microbiol. 149, 1753–1761
39 Vazquez-Laslop, N. et al. (2001) Molecular sieve mechanism of
26 Bayer, M.H. and Keck, W. (1990) Localization of penicillin-binding
selective release of cytoplasmic proteins by osmotically shocked
protein 1b in Escherichia coli: immunoelectron microscopy and
Escherichia coli. J. Bacteriol. 183, 2399–2404
immunotransfer studies. J. Bacteriol. 172, 125–135
40 Prats, R. and De Pedro, M.A. (1989) Normal growth and division of
27 Ishidate, K. et al. (1986) Isolation of differentiated membrane domains
Escherichia coli with a reduced amount of murein. J. Bacteriol. 171,
from Escherichia coli and Salmonella typhimurium, including
Elsevier celebrates two anniversaries with
gift to university libraries in the developing world
In 1580, the Elzevir family began their printing and bookselling business in the Netherlands, publishing works by scholars such as JohnLocke, Galileo Galilei and Hugo Grotius. On 4 March 1880, Jacobus George Robbers founded the modern Elsevier company intending,just like the original Elzevir family, to reproduce fine editions of literary classics for the edification of others who shared his passion, other'Elzevirians'. Robbers co-opted the Elzevir family's old printer's mark, visually stamping the new Elsevier products with a classic oldsymbol of the symbiotic relationship between publisher and scholar. Elsevier has since become a leader in the dissemination ofscientific, technical and medical (STM) information, building a reputation for excellence in publishing, new product innovation andcommitment to its STM communities.
In celebration of the House of Elzevir's 425th anniversary and the 125th anniversary of the modern Elsevier company, Elsevier will donatebooks to 10 university libraries in the developing world. Entitled ‘A Book in Your Name', each of the 6 700 Elsevier employees worldwidehas been invited to select one of the chosen libraries to receive a book donated by Elsevier. The core gift collection contains thecompany's most important and widely used STM publications including Gray's Anatomy, Dorland's Illustrated Medical Dictionary,Essential Medical Physiology, Cecil Essentials of Medicine, Mosby's Medical, Nursing and Allied Health Dictionary, The Vaccine Book,Fundamentals of Neuroscience, and Myles Textbook for Midwives.
The 10 beneficiary libraries are located in Africa, South America and Asia. They include the Library of the Sciences of the University ofSierra Leone; the library of the Muhimbili University College of Health Sciences of the University of Dar es Salaam, Tanzania; the libraryof the College of Medicine of the University of Malawi; and the libraries of the University of Zambia, Universite du Mali, UniversidadeEduardo Mondlane, Mozambique; Makerere University, Uganda; Universidad San Francisco de Quito, Ecuador; Universidad FranciscoMarroquin, Guatemala; and the National Centre for Scientific and Technological Information (NACESTI), Vietnam.
Through ‘A Book in Your Name', the 10 libraries will receive approximately 700 books at a retail value of approximately 1 million USdollars.
For more information, visit www.elsevier.com
Source: http://toukach.ru/files/tim_mur3.pdf
RHS Science developments RHS Science Strategy By sharing and improving RHS horticultural research the new Science Strategy aims (via four key themes) to continue to guide gardeners and growers. Using the vast, ever-growing collections of plants, insects, pathogens, books and art in its possession, the RHS can maintain its place as a leading contributor
Case Report Chronic Osteomyelitis ina Newborn Paediatrics Section BaPPaditya daS, PranaB Kumar dey, SatyaBrata roy chowdhury, KalPana datta showed features of chronic osteomyelitis. A diagnosis A term, 14-day-old male baby was presented with high of chronic osteomyeltis with septicaemia was made grade fever, decreased feeding, lethargy, progressively and treated conservatively with intravenous antibiotics