Doi:10.1016/j.mcn.2005.11.01
Mol. Cell. Neurosci. 31 (2006) 574 – 585
NMDA receptors mediate calcium-dependent, bidirectional changes indendritic PICK1 clustering
K.G. Sossa, B.L. Court, and R.C. Carroll*
Department of Neuroscience, Rose Kennedy Center for Mental Retardation, 1410 Pelham Parkway,Albert Einstein College of Medicine, Bronx, NY 10461, USA
Received 16 August 2005; revised 7 November 2005; accepted 22 November 2005Available online 10 January 2006
AMPA receptor (AMPAR) trafficking at CNS synapses is regulated by
rearrangements underlying synaptic depression (
several receptor-binding proteins. One model of AMPAR endocytosis
Malenka, 2002).
entails the cotargeting of the GluR2-interacting protein PICK1 and
Several studies have established a requirement for GRIP/ABP
activated PKC to synapses. We demonstrate that NMDA receptor
and/or PICK1 binding to GluR2 in the regulated endocytosis of
(NMDAR) activation mediates bidirectional changes in surface
AMPARs, which mediates LTD in the hippocampus and cerebellum
AMPARs through two additional forms of PICK1 redistribution. In
neurons, NMDAR activation, which induces AMPAR endocytosis,
2000). In one prominent scheme for this process, GRIP/ABP, via a
increases endosomal PICK1 clustering. In contrast, stronger NMDARactivation rapidly reduces PICK1 clustering accompanied by decreases
PDZ domain, anchors AMPARs at synaptic membranes (
in PICK1/GluR2 association and increases in surface AMPAR levels.
2003), while PICK1, binding to the same C-terminal site of GluR2,
PICK1-siRNA similarly increases surface AMPARs and occludes the
can mediate the mobilization of AMPARs. For example, PICK1
NMDAR-mediated effect, demonstrating the role of PICK1 in this
interacts with activated C-protein kinase a (PKCa) and synaptically
process. Bidirectional NMDAR-mediated changes in PICK1 localiza-
localizes the kinase, enabling phosphorylation of GluR2-Ser880
tion are determined by the magnitude of receptor-activated dendritic
(This phosphorylation decreases GRIP-GluR2
calcium signals. Our results show that PICK1 localization in dendrites
association and facilitates AMPAR internalization (
is subject to multiple forms of regulation that contribute to surface
1999; Chung et al., 2000; Perez et al., 2001). A recent report further
AMPAR expression, likely by modulating the numbers of AMPARs
demonstrates that the direct interaction between GRIP/ABP and
maintained in intracellular compartments.
D
PICK1 is important for Ser880 phosphorylation and GluR2
2005 Elsevier Inc. All rights reserved.
trafficking (Although this evidence provides acompelling model for regulated AMPAR endocytosis, questionsremain as to how signaling pathways that drive receptor endocytosis
in the absence of PKC activation modulate PICK1.
The role of PICK1 in AMPAR trafficking has proven more
Neurotransmission at many CNS synapses is regulated by the
complex than simply to enhance receptor endocytosis. Knockout
dynamic trafficking of AMPA-type glutamate receptors (AMPARs)
mice revealed PICK1's importance in the recycling of AMPARs to
into and out of the membrane surface. The modulation of this
the membrane surface in cerebellar stellate cells (
process occurs via the interaction of AMPAR subunits (i.e. GluR1 –
2005). Disruption of GRIP and PICK1 interaction interrupts the
4) and cytosolic proteins. In recent years, much attention has been
recycling of AMPARs in hippocampal neurons (
given to the protein – protein interactions of GluR2-containing
As GRIP/ABP may be targeted to intracellular as well as synaptic
AMPARs, which have been shown to influence both surface
membranes (recycling may be dependent on the
receptor maintenance and activity-driven changes in synaptic
PICK1-mediated disruption of internalized AMPARs stabilized by
strength (In particular, investigations of the
GluR2 cytoplasmic tail interacting proteins glutamate receptor
interacting protein (GRIP/ABP) and PICK1 protein interacting with
PICK1 itself may play a role in stabilizing receptors away from
C-kinase have brought a closer understanding of the synaptic
surface synaptic sites. The disruption of the interaction of PICK1and GluR2 with intracellularly introduced peptides results in therapid delivery of AMPARs to synapses (
* Corresponding author. Fax: +1 718 430 2721.
E-mail address: (R.C. Carroll).
et al., 2004). Additionally, overexpression of PICK1 causes a
Available online on ScienceDirect
reduction in the surface levels of GluR2 (
1044-7431/$ - see front matter D 2005 Elsevier Inc. All rights reserved.
doi
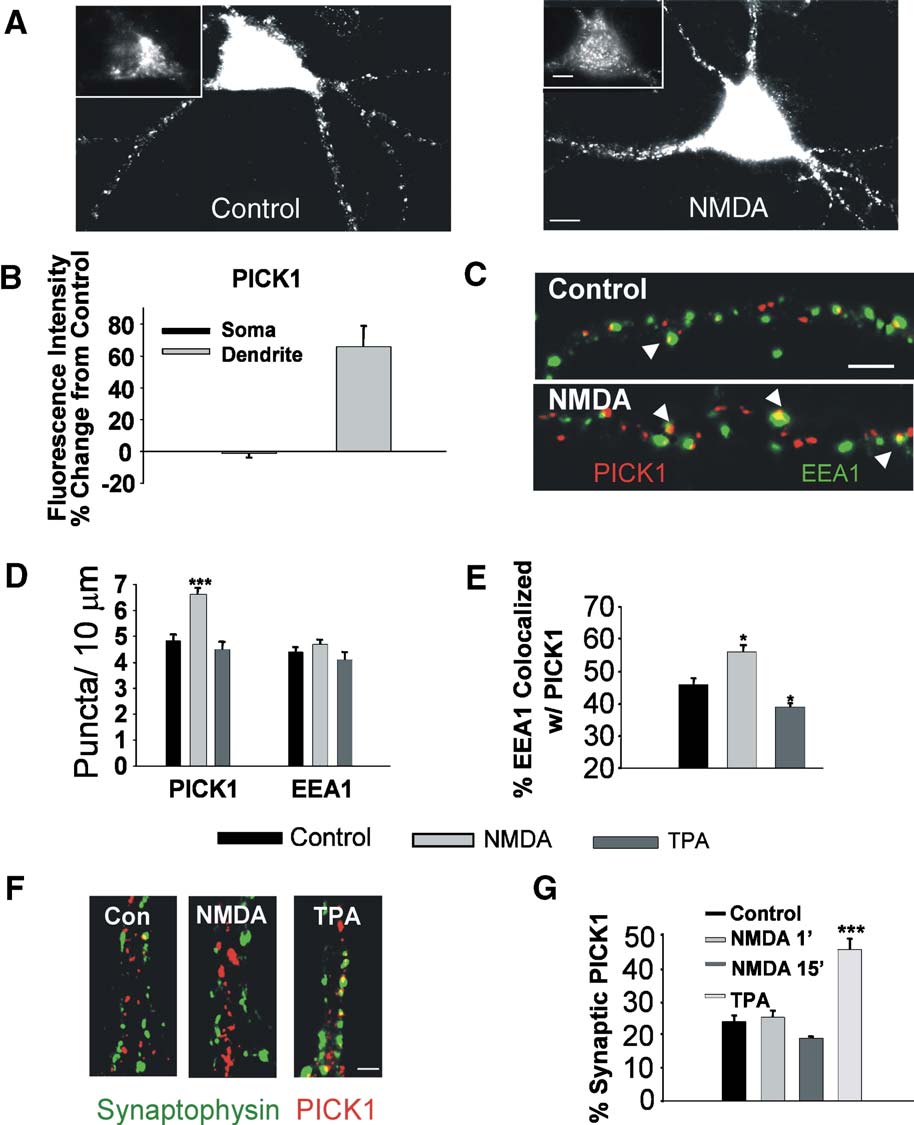
K.G. Sossa et al. / Mol. Cell. Neurosci. 31 (2006) 574 – 585
Together, these results suggest that PICK1 is retaining GluR2-
The functions proposed for PICK1 in AMPAR delivery,
containing AMPARs away from the synapse. It remains to be
recycling, and intracellular stabilization suggest the importance
determined whether the interaction of PICK1 and GluR2 can be
of localizing PICK1 to appropriate compartments. The ability of
modulated by the activation of cellular signaling pathways as a
PICK1 to mediate the destabilization of GRIP-bound AMPARs
means for elevating surface AMPARs. Interestingly, both electro-
from synaptic or intracellular compartments necessitates the
physiological (and imaging (
selective targeting of PICK1 to these sites. Furthermore, if PICK1
analyses have demonstrated that NMDA-type glutamate receptor
mediates the stabilization of a population of intracellular AMPARs,
(NMDAR) signaling can trigger the synaptic insertion of previously
its clustering with these receptors must be subject to regulation. In
internalized AMPARs: a population of receptors modulated by
this way, mechanisms that regulate the subcellular distribution and
clustering of PICK1 could locally and bidirectionally influence
Henley, 2003). Thus, NMDAR activation may alter GluR2-PICK1
functional AMPAR levels.
association in order to increase as well as decrease surface AMPAR
Here, we show that the extent and localization of PICK1
clustering are modulated by receptor signaling pathways. Activa-
Fig. 1. NMDA receptor stimulation enhances dendritic PICK1 clustering in endosomal but not in synaptic compartments. (A) Imaged neurons immunolabeledfor PICK1 show strong somatic (inset) and punctate dendritic staining (left). NMDA treatment (50 AM, 2 min) results in increased dendritic PICK1 labeling(right). (B) Quantitation of the intensity of PICK1 labeling in soma and dendrites of NMDA treated neurons. Values are presented as a percent of control. (C)Imaged dendrites of neurons colabeled for PICK1 (red) and EEA1 (green). NMDA activation causes increased dendritic PICK1 puncta including thosecolocalized with EEA1 (arrowheads). (D) Quantitation of dendritic PICK1, and EEA1 puncta per unit length in untreated control, NMDA-treated (50 AM, 1min), and TPA treated (100 nM, 15 min) neurons. (E) Quantitation of percent of EEA1 puncta colocalized with PICK1 in control, NMDA-, and TPA-treatedneurons. (F) Dendrites immunolabeled for PICK1 (red) and synaptophysin (green) are shown for control, NMDA, and TPA treated neurons. (G) The percent ofPICK1 clusters localized at synapses was quantified for neurons that were untreated (control), NMDA-treated for 1 min and immediately fixed, NMDA-treatedfor 1 min and fixed after 15 min, and TPA-treated for 15 min. Phorbol esters induce an increase in the synaptic localization of PICK1, as reported previously,while NMDA has no effect. Scale bar: A, 5 AM; C, 3 AM. *P < 0.05, ***P < 0.001.
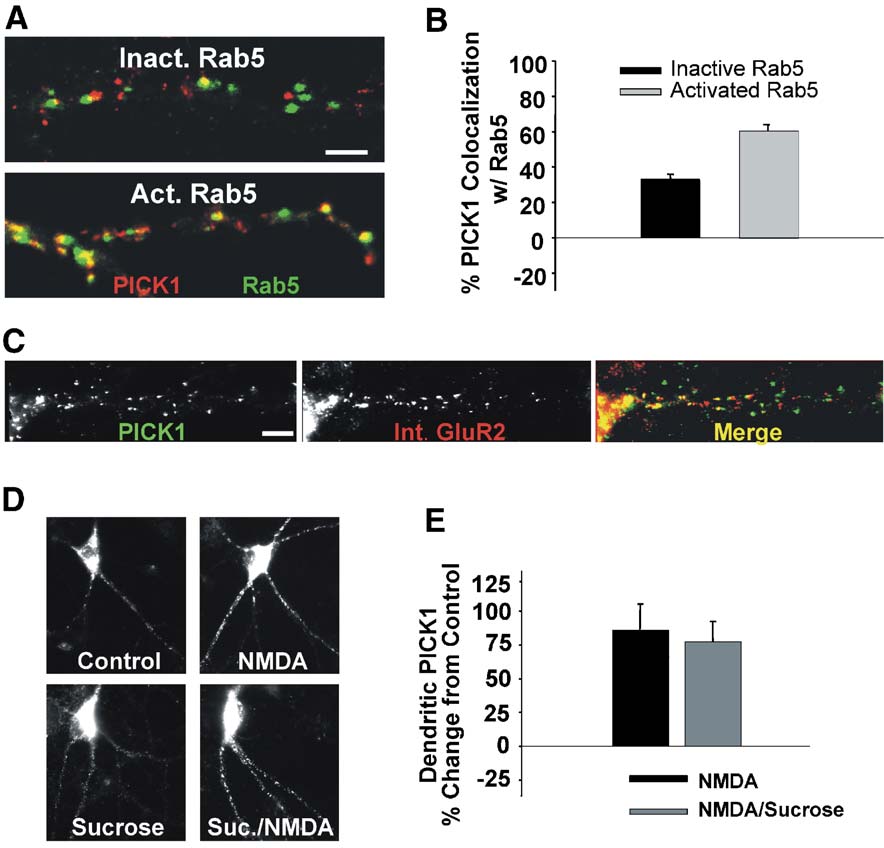
K.G. Sossa et al. / Mol. Cell. Neurosci. 31 (2006) 574 – 585
tion of NMDARs triggers rapid, bidirectional changes in PICK1
PICK1 similar to that induced by activation of PKCa (
clustering in dendritic endosomes, differing from the phorbol ester-
2000; Perez et al., 2001). In cultured hippocampal neurons, 2 min
activated translocation of PICK1 to synaptic sites. Moderate
exposure to NMDA (resulted in an increase in
NMDAR signaling increases PICK1 clustering in dendritic endo-
the dendritic immunolabeling of PICK1 after 15 min, both in
somes. In contrast, stronger NMDAR activation decreases den-
overall intensity and in number of puncta (B, D, 64 T
dritic PICK1 clustering. Interestingly, the robust activation of
13% increase in intensity, control 4.9 T 0.14 PICK1 clusters/10 Am,
NMDARs, which reduces PICK1/GluR2 interactions, increases
NMDA 6.6 T 0.18 clusters/10 Am, n = 3, P < 0.001).
dendritic surface AMPAR expression, demonstrating the impor-
PICK1 clusters were found largely colocalized with transferrin-
tance of this process in modulating AMPAR trafficking. These
positive as well as with EEA1-labeled early endosomes (76.1 T 3.1%
differential NMDAR-mediated effects can be attributed to dendritic
PICK1 vesicles colocalized with transferrin, data not shown;
calcium levels activated under each condition: large calcium
1C, 43.9 T 3.5% PICK1 vesicles colocalized with EEA1). The
signals drive a reduction in PICK1, while smaller calcium signals
numbers of EEA1 positive clusters were unchanged following
induce increases in PICK1. Our results suggest that NMDARs can
NMDA treatments (However, the proportion of transferrin
bidirectionally regulate the clustering of PICK1 at endosomes,
and EEA1 clusters colocalized with PICK1 was enhanced by
thereby modulating the retention of intracellular AMPARs and
treatment with the NMDA (transferrin: control 67.3 T 3.6%,
consequently affecting surface AMPARs levels.
colocalized, NMDA 82.1 T 2.3% n = 4, P < 0.05, data not shown;EEA1: control 47.7 T 2.0%, NMDA 56.1 T 2.1%, n = 3, P <0.05). In contrast, 15-min treatment with phorbol esters did not
increase the numbers of dendritic PICK1 puncta (4.5 T 0.34puncta/10 Am), and decreased the extent of EEA1 vesicles
NMDAR activation reduces dendritic PICK1 clustering
colocalized with PICK1 (phorbol ester 39.4 T 1.3%colocalized, n = 4, P < 0.05), indicating that NMDAR and PKC
We initially tested whether or not activation of NMDARs,
activation has differential effects on the localization of dendritic
which induces AMPAR endocytosis, causes a relocalization of
Fig. 2. NMDA-induced PICK1 clustering occurs preferentially at vesicles expressing activated rab5 and does not require endocytosis. (A) PICK1 clusterstogether preferentially with activated but not inactivated rab5. Neurons transfected with activated (Q79L) or inactivated (S34N) rab5-GFP (green) were fixedafter 36 h and immunolabeled for PICK1 (red). (B) Quantitation of the percent of PICK1 clusters colocalized with either inactivated or activated rab5. (C)PICK1 is coclustered with internalized AMPARs. Live neurons were labeled with an anti-N terminal GluR2 antibody and subsequently treated with NMDA toinduce AMPAR internalization. Following fixation, PICK1 (left) and internalized AMPARs (center) were immunocytochemically detected. Merge (right)demonstrates coclustering of internalized receptors with PICK1. (D) Blockade of clathrin-mediated endocytosis by hypertonicity (350 mM sucrose duringtreatment) does not block the NMDA-induced increase in PICK1 clustering. (E) Quantitation of the effects of hypertonic extracellular media on the NMDA-mediated changes in dendritic PICK1 levels. Scale bars: A and C, 3 AM.
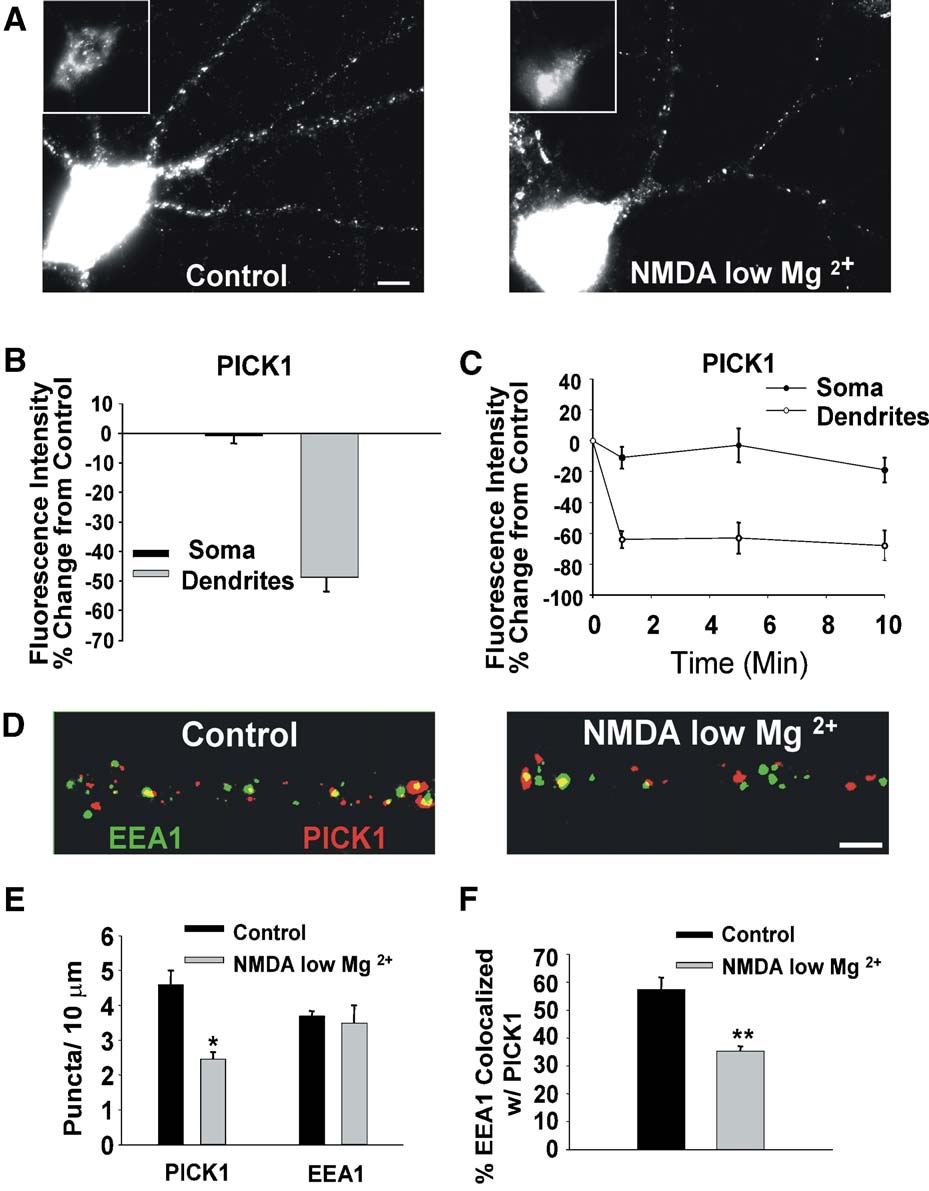
K.G. Sossa et al. / Mol. Cell. Neurosci. 31 (2006) 574 – 585
Fig. 3. Increased NMDAR activation stimulates rapid unclustering of dendritic PICK1. (A) Enhanced activation of NMDARs by treating with NMDA (50 AM,1 min) in reduced extracellular magnesium (0.13 mM) induces a reduction in dendritic PICK1 clustering 15 min after treatment. Representative images areshown of PICK1 immunolabeled neurons that were either untreated (left) or NMDA/low Mg2+-treated (right). Insets show soma with a brightness level toenable visualization of labeling. (B) Quantitation of the intensity of PICK1 labeling in soma and dendrites of NMDA/low Mg2+-treated neurons. Values arepresented as percent of control. (C) Time course demonstrates that NMDA/low Mg2+ treatment produces considerable changes in dendritic PICK1 within 1min, which stabilizes after 5 min. Somatic labeling shows little change. (D) Imaged dendrites of neurons colabeled for PICK1 (red) and EEA1 (green). NMDA/low Mg2+ activation causes decreased dendritic PICK1 puncta, with a lower proportion colocalized with EEA1 (arrowheads). (E) Quantitation of dendriticPICK1, and EEA1 puncta per unit length in untreated control and NMDA/low Mg2+-treated (50 AM, 1 min) neurons. (F) Quantitation of percent of EEA1puncta colocalized with PICK1 in control and NMDA/low Mg2+-treated neurons. Scale bar: A, 5 Am, **P < 0.01, *P < 0.05.
Similar to previous studies (
activation rapidly increases the dendritic clustering of PICK1
2001), we found that phorbol ester treatment (100 nM TPA, 15
including its association with early endosomes, while PKC
min) resulted in an increased localization of PICK1 at synaptic
activation redistributes PICK1 from endosomes to synaptic sites.
sites (, G, control 23.7 T 1.8%, TPA 45.2 T 3.1% PICK1clusters colocalized with synaptophysin, n = 5, P < 0.001). NMDA
PICK1 clustering in endosomes is influenced by the activation
treatment, however, had no effect on the proportion of PICK1
puncta at synapses after 15 min (, G, 18.7 T 0.6%colocalized, n = 4). Even 1 min after NMDA treatment, there was
As rab5 is localized at early endosomes where PICK1
an increase in the number of dendritic PICK1 puncta (control 4.1 T
accumulates, and is activated by NMDAR stimulation (
0.11, NMDA 1 min 5.1 T 0.11 puncta/10 Am, n = 5), with no effect
al., 2005), we tested whether the activation state of rab5 could
on the proportion of PICK1 at synapses (24.9 T 1.9%
affect the localization of PICK1. Hippocampal neurons were
colocalized, n = 5). Together, these data suggest that NMDAR
transfected either with an activated (GTP hydrolysis deficient,
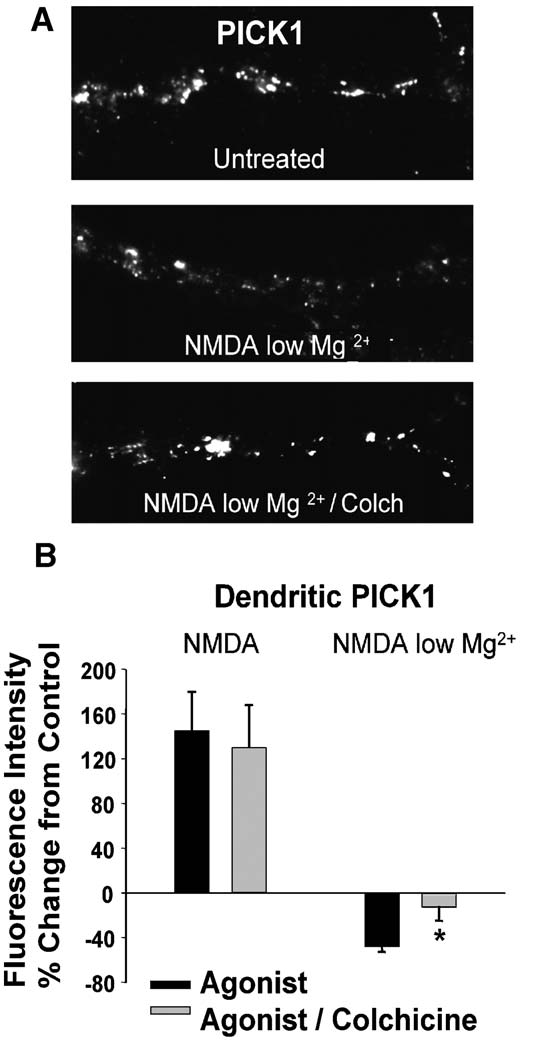
K.G. Sossa et al. / Mol. Cell. Neurosci. 31 (2006) 574 – 585
Q79L) or an inactivated (low affinity for GTP, S34N) form of rab5(and after 36 h were immunolabeled forPICK. In transfected neurons, 61.0 T 4% of PICK1 clusters werecolocalized with the activated form of rab5 while only 33.5 T 2%of PICK1 colocalized with the inactive form (B).
Dendritic PICK1 accumulation occurs independently of AMPARendocytosis
Both NMDAR and rab5 activations are known to increase
AMPAR endocytosis (Furthermore, we found that NMDAR signaling induces acoclustering of internalized AMPARs with PICK1 in dendrites(We, therefore, examined whether the clustering ofPICK1 is dependent on its association with internalized AMPARs.
Hypertonic media (350 mM sucrose) block clathrin-mediatedendocytosis (and have been shown to inhibitglutamate receptor-mediated AMPAR internalization (1999). Incubating neurons in hypertonic media during NMDAtreatment did not block the increased clustering of PICK1 (2D, E, NMDA 83.6 T 21.2% increase from control, NMDA/sucrose 77.5 T 13.7%). This result indicates that the accumulationof internalized AMPARs is not necessary for NMDAR-mediatedPICK1 clustering.
Strong NMDAR activation drives the loss of clustered dendriticPICK1
We further sought to determine whether activity that might
drive the insertion of AMPARs would have any effect ondendritic PICK1 levels. Traditional models of NMDAR plastic-ity suggest that while weaker activation of NMDARs triggersLTD, stronger activation of NMDARs couples to LTP or de-depression, presumably through increased calcium influx (man, 1989). We therefore enhanced the activation of NMDARs by
Fig. 4. NMDA/low Mg2+-induced loss of PICK1 levels requires
applying the agonist (1 min) in low magnesium ACSF (NMDA/
microtubules. (A) Labeling of PICK1 in cells treated T colchicine (10
low Mg2+, 0.13 mM Mg2+) to reduce the extracellular blockade of
AM for 30 min) prior to NMDA/low Mg2+-exposure. (B) Quantitation
the channel by magnesium. Following NMDA/low Mg2+ treat-
of colchicine's effects on NMDA- and NMDA/low Mg2+-induced
ment, we observed a marked decrease in the dendritic labeling of
changes in dendritic PICK1 labeling. The NMDA/low Mg2+-meditated
PICK1 (Changes were observed both in the overall
reduction, but not the NMDA-dependent increase in PICK1 labeling is
labeling intensity and in the number of dendritic puncta (
E, intensity: soma
0.8 T 2.6%, dendrites
48.7 T 4.8%, n = 14,
puncta: control 4.6 T 0.4%, NMDA/low Mg2+ 2.5 T 0.6 puncta/10Am, n = 3, P < 0.05). The changes were rapid and near maximal
0.05). This result is consistent with a role for microtubule-
within 1 min of agonist exposure (The lower PICK1
dependent vesicle trafficking in the unclustering of PICK1 in
clustering reduced the extent of EEA1 positive endosomes
coclustered with PICK1 (F, control 57.5 T 4.2%, NMDAlow Mg2+ 35.4 T 4.6% colocalized, n = 3, P < 0.01).
Increased NMDAR activation reduces the association of GluR2and PICK1
Regulated PICK1 loss in dendrites is microtubule-dependent
The unclustering of PICK1 in hippocampal dendrites suggests
The later steps of endosome processing, including sorting, are
that the activation of the NMDAR results in the uncoupling of
dependent on microtubules (There-
PICK1 from GluR2 at endosomes. We tested this biochemically in
fore, we tested whether the redistribution of PICK1 away from
untreated and NMDA/low Mg2+-treated hippocampal slices.
endosomes requires microtubule-based transport. NMDAR-in-
Lysates were immunoprecipitated with GluR2 antibodies, and
duced PICK1 clustering was not inhibited by the pretreatment of
assayed for PICK1 association by Western blot. Probing with anti-
cultures with colchicine (30 min pretreatment, 10 AM;
PICK1 antibodies demonstrated a significant decrease ( 25.4 T
NMDA, 145.1 T 34.5%, NMDA/colchicine, 105.8 T 33.0%, n = 4).
6.9% change from untreated control, n = 4, P < 0.05) of GluR2/
However, colchicine treatment eliminated NMDA/low Mg2+-
PICK1 association in NMDA/low Mg2+-treated slices (
induced loss of PICK1 (B, NMDA/low Mg2+,
Immunoprecipitation with a nonspecific mouse IgG revealed no
5.5%, NMDA/low Mg2+/colchicine,
12.6 T 11.9%, n = 4, P <
staining for PICK1 (
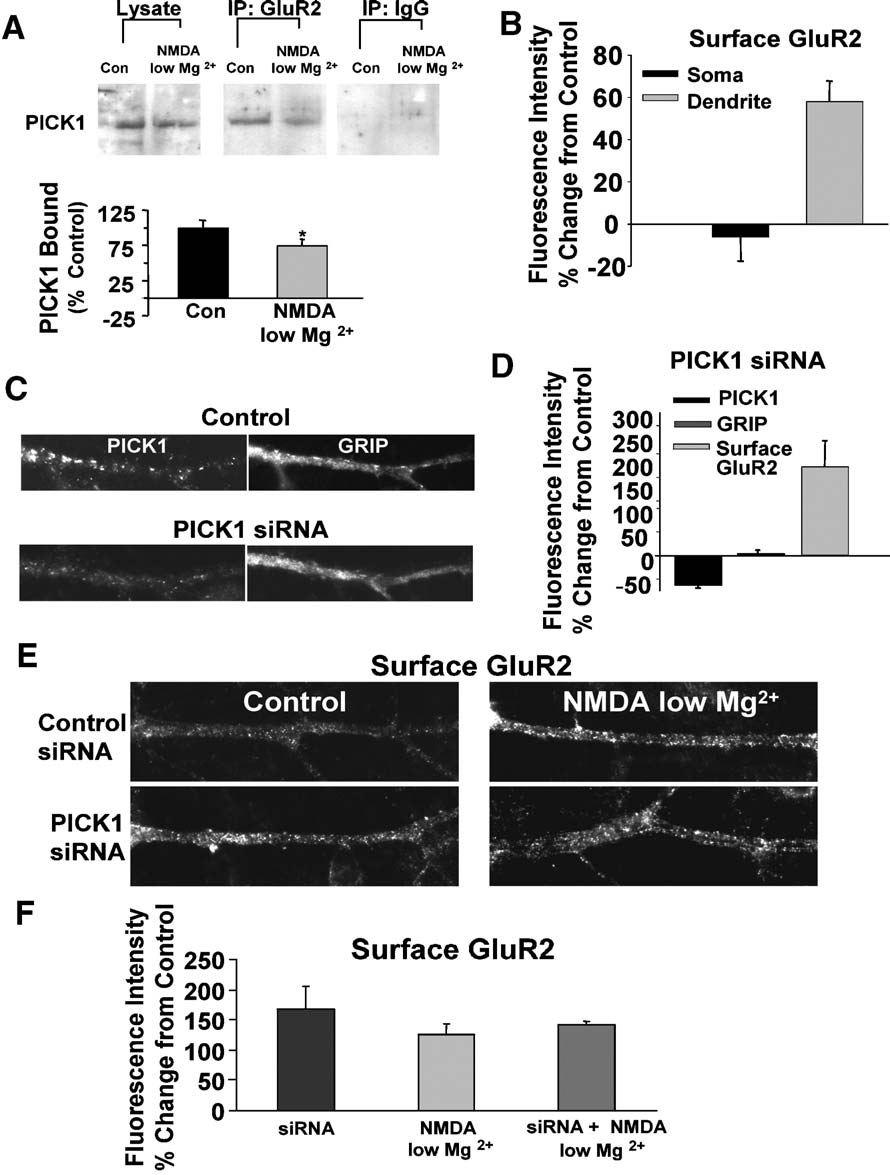
K.G. Sossa et al. / Mol. Cell. Neurosci. 31 (2006) 574 – 585
Fig. 5. NMDA/low Mg2+ induces a PICK1-dependent increase in surface GluR2-containing AMPARs. (A) NMDA/low Mg2+ treatment reduces PICK1/GluR2interaction in hippocampal slices. Lysates from treated and untreated hippocampal slices were immunoprecipitated with an anti-GluR2 or a nonspecific mouseIgG antibody. Total lysates and immunoprecipitated proteins were subjected to SDS-PAGE and probed by Western blot for PICK1 (top). Quantitation of PICK1coimmunoprecipitated with GluR2 (bottom) shows a reduction in PICK1/GluR2 association with NMDA/low Mg2+ treatment. The ratio of immunoprecipitatedPICK1 to total PICK1 levels in the lysate was determined, and is presented as a percent of control (n = 4, *P < 0.05, paired Student's t test). (B) Quantitation ofthe intensity of surface GluR2 immunocytochemical labeling demonstrates an increase in surface receptor levels following NMDA/low Mg2+ treatment.
Somatic GluR2 levels show little change while dendrites exhibit a large increase. (C) Introduction of PICK1-siRNA mediates a decrease inimmunocytochemically detected PICK1 (left panels) in hippocampal neurons compared to neurons treated with scrambled siRNA. Levels of GRIP1 (rightpanels) were unaffected. (D) PICK1 – siRNA decreases dendritic PICK1 and increases surface GluR2 levels. The graph depicts analysis of the intensities ofimmunocytochemically detected PICK1, GRIP, and surface GluR2 in PICK1-siRNA versus scrambled siRNA-treated neurons (PICK1 and GluR2, n = 6;GRIP, n = 3). (E) Immunolabeling of surface GluR2 in control (left) and NMDA/low Mg2+-treated (right) neurons. Cells were exposed to control scrambledsiRNA (top) or PICK1 – siRNA bottom. Control siRNA cells treated with NMDA/low Mg2+ show an increase in surface GluR2 labeling. PICK1-siRNA cellsexhibit higher basal surface GluR2 levels that are not further enhanced by NMDA/low Mg2+ treatment. (F) Quantitation of siRNA experiments shown in panelE. Results are presented as percent change in fluorescent intensity of GluR2 labeling from control. *P < 0.05.
Reduction of clustered PICK1 in dendrites mediates an increase in
increase in surface dendritic GluR2s (58.1 T 10.5%
surface AMPAR expression
increase from control, n = 7, P < 0.001). GluR1 receptor levelsincreased in a similar manner although with a lesser magnitude of
To test whether NMDAR-mediated reduction in PICK1
change (40.8 T 8.2% increase from control, n = 4, P < 0.05, data
clustering and GluR2 binding directly influences surface
AMPARs, we measured changes in surface AMPARs following
The increase in surface AMPARs following NMDA/low Mg2+
strong activation of NMDARs. Immunocytochemical analysis
treatment is consistent with, though not necessarily the direct result
demonstrated that NMDA/low Mg2+ treatment results in an
of, PICK1 redistribution. To test the role of reducing dendritic
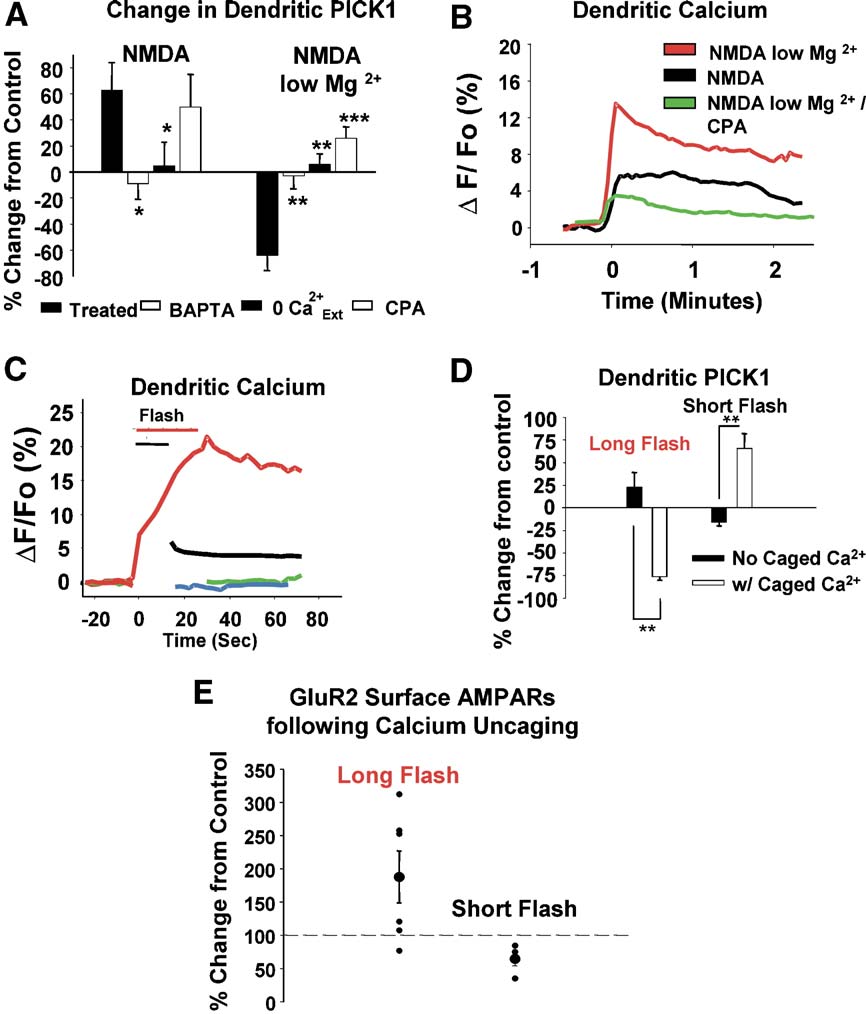
K.G. Sossa et al. / Mol. Cell. Neurosci. 31 (2006) 574 – 585
PICK1 on surface AMPARs, we used an RNAi approach to
as compared to cells treated with control-siRNA, but to a lesser
knockdown the expression of PICK1. After treatment with PICK1-
extent (52.0 T 20.5% change from control, n = 5, data not shown).
siRNA for 72 h, immunocytochemically detected PICK1 was
To test whether this PICK1-mediated change in surface GluR2
reduced to 36 T 4% (n = 6, P = 0.01) of levels in cultures loaded
is the likely mechanism behind the increase in surface AMPARs
with a control scrambled-siRNA (D). Levels of the
following strong NMDAR activation, we applied NMDA/low
AMPAR interacting protein GRIP1, in contrast, were unaffected
Mg2+ to siRNA treated cells (F). While in control-
indicating the specificity of the siRNA effect (+2.8 T 6.0%, n = 4
siRNA-treated cells there was an increase in surface AMPAR
ns, D). Analysis of siRNA treated neurons showed that
following treatment (126.5 T 17.4%, n = 4), siRNA-treated cells
the reduction of PICK1 levels was also accompanied by a
showed no further increases in surface receptor levels following
significant increase in surface GluR2-containing AMPARs (
NMDA/low Mg2+ treatment ( 9.1 T 12.7% siRNA-NMDA/low
5D, E, 168.4 T 37% change from control, n = 6, P < 0.05). Surface
Mg2+-treated/siRNA alone, n = 4). This occlusion supports the
GluR1 levels were also higher in cells treated with PICK1-siRNA
conclusion that the loss of dendritic PICK1 with NMDA/low Mg2+
Fig. 6. Bidirectional receptor-induced changes in PICK1 clustering are determined by the magnitude of intracellular calcium signals. (A) NMDA/low Mg2+-and NMDA-mediated changes in PICK1 are blocked in neurons loaded with BAPTA-AM (25 AM) or treated in the absence of extracellular calcium (0 Ca2+
Prevention of intracellular calcium release with CPA (25 AM) reveals that the effect of NMDA/low Mg2+ treatment, and not that of NMDA treatment, isdependent on the release of calcium from intracellular stores. (B) Fluo-3-AM (1 AM) calcium imaging of hippocampal neurons treated with NMDA/low Mg2+ TCPA (black and red, respectively), and NMDA in regular (green). NMDA/Low Mg2+ produces a large calcium response, while lower calcium signals areevident for NMDA alone and NMDA/low Mg2+/CPA. (C) Photolytic uncaging of caged calcium generates elevations in dendritic calcium comparable to thoseelicited by activation of receptors with NMDA/low Mg2+ and NMDA. Hippocampal neurons loaded with o-nitrophenyl-EGTA-AM were stimulated with either7 (red) or 15 (black) 2 s flashes of dim UV light to elicit small or large calcium responses as measured by fluo-3-AM fluorescence. Cells loaded with Fluo-3-AM alone show no changes in calcium following stimulation with 7 (green) or 15 (blue) flashes. (D) Stimulation with 15 flashes (long) results in a markeddecrease in dendritic PICK1 levels, while the short stimulation (7 flashes) results in increased dendritic PICK1 labeling. In the absence of caged calcium, thereis little effect on PICK1 levels. (E) The magnitude of cytosolic calcium levels differentially influences surface GluR2 levels in dendrites. Neurons weresubjected to photolytic uncaging of calcium as described. Five minutes following uncaging flash, neurons were fixed and labeled for surface GluR2. Exposureto a long flash that elicits a large calcium elevation resulting in a net, albeit variable, increase in surface AMPARs (+88 T 40%, n = 6). Neurons from five of sixexperiments showed an increase in surface AMPARs. A short flash, which elicited a smaller calcium response, resulted in a decrease in surface AMPARs( 46 T 10%, n = 4 compared to unflashed controls). *P < 0.05, **P < 0.01, ***P < 0.001.
K.G. Sossa et al. / Mol. Cell. Neurosci. 31 (2006) 574 – 585
activation accounts for the observed receptor-mediated increases in
of control levels, n = 9). Control flashes in the absence of loaded
surface AMPARs.
calcium cage showed no net change in basal PICK1 levels (6C, D). These data demonstrate that the bidirectional regulation of
Differential PICK1 redistribution is calcium-dependent
dendritic PICK1 clustering is dependent on the magnitude ofcytosolic calcium signals. Consistent with a link between changes
The triggering of bidirectional NMDAR-dependent plasticity has
in the clustering of dendritic PICK1 and surface AMPAR
been suggested to be determined by extent of calcium entry through
expression, higher levels of uncaged calcium resulted in an
the channel: small elevations in cytosolic calcium drive depression,
increase in surface AMPAR levels in 4 of 6 experiments, whereas
and large increases induce potentiation (
lower calcium levels reduced surface AMPARs (4 of 4
1999). Therefore, we investigated whether calcium signals might be
responsible for the opposing changes seen in the dendritic clusteringof PICK1. Both the NMDAR-mediated increases and decreases indendritic PICK1 were blocked by preincubating cultured neurons
with a cell permeable calcium chelator, BAPTA-AM (30 minpretreatment, 25 AM; NMDA, 62.7 T 21.4%, NMDA/
We report here, for the first time, that levels of clustered PICK1
10.4 T 5.8%, n = 4, P < 0.05, NMDA/low Mg2+, 63.6 T
in dendrites can be directly modulated by the activation of a
10.9%, NMDA/low Mg2+/BAPTA,
2.7 T 9.6%, n = 4, P < 0.01).
neurotransmitter receptor. We find that moderate NMDAR
Similarly, removal of extracellular calcium blocked the effects,
stimulation increases the clustering of PICK1 in dendrites. In
supporting the possibility that calcium influx through the NMDARs
contrast, strong NMDAR activation drives the loss of dendritic
mediates the changes (NMDA/0 Ca2+
ext 4.7 T 18%, n = 5, P <
PICK1 clusters, via microtubule-dependent relocalization. This
0.05, NMDA/low Mg2+/0 Ca2+
ext 6.1 T 8.5%, n = 4, P < 0.01). We also
differential effect of NMDAR activation on PICK1 clustering is
investigated whether the release of calcium from intracellular stores
directly dependent on the magnitude of the calcium response
plays a role in PICK1 redistribution. We found that while the
generated in the dendrites. As PICK1 is implicated in AMPAR
preincubation of cultured neurons with cyclopiazonic acid (CPA; an
trafficking, its regulation by NMDAR activation could be expected
inhibitor of intracellular calcium ATPases) had no effect on
to impact the expression of surface dendritic AMPARs. Consistent
NMDAR-mediated increases in dendritic PICK1, this treatment
with this, moderate NMDAR stimulation, which results in PICK1
blocked, and partially reversed, the NMDA/low Mg2+-mediated
accumulation in dendrites, is known to decrease surface AMPAR
reductions in PICK1 (NMDA, 50.2 T 25.5%, NMDA/low
levels (Strong NMDAR activation, on the other
Mg2+/CPA, +26.1 T 7.9%). This suggests that the calcium influx
hand, couples to increases in surface dendritic AMPARs via a
through NMDARs under conditions of strong receptor activation
PICK1-dependent process. Collectively, our data provide evidence
results in calcium-induced calcium release that contributes to the
that dendritic PICK1 clustering is bidirectionally regulated and
loss of PICK1 labeling in dendrites.
influences local levels of surface AMPARs, likely by modulating
Since both increases and decreases in PICK1 can be mediated
the numbers of receptors found in intracellular pools.
by NMDAR-induced changes in calcium, we sought to measure
Previous studies showed that phorbol esters and basal PKC
the calcium responses that mediate these effects. In fluo-3-AM
activity induce a synaptic localization of PICK1 and decreased
loaded neurons, NMDA induced a moderate increase in the
surface AMPAR levels (
dendritic levels of calcium (However, NMDA applied
Terashima et al., 2004). We likewise see in our system that PKC
in low extracellular magnesium induced a response nearly twice
activation leads to a shift in the localization of PICK1 clusters from
the size (The same treatment in the presence of CPA
early endosomes to synaptic sites without an increase in the overall
induced a calcium response slightly smaller than the NMDA
number of dendritic PICK1 clusters. It has been suggested that
PICK1, under these conditions, may be important for triggering the
These results raise the question of whether the magnitude or the
events that initialize AMPAR endocytosis, including the facilita-
source of calcium may dictate the differential effects on PICK1
tion of PKC-mediated GluR2 phosphorylation. We find that a
localization. To directly test whether calcium levels alone may
second pathway linked to AMPAR endocytosis, NMDAR activa-
influence the distribution of dendritic PICK1, we employed
tion, has a contrasting effect on dendritic PICK1. Activation of this
photolytic uncaging of calcium to directly manipulate cytosolic
pathway leads to an increased number of PICK1 clusters in
levels of the ion and monitor its effect on PICK1. Dim light
dendrites without an evident shift towards synaptic sites. While it is
stimulation of neurons loaded with caged calcium (o-nitrophenyl-
possible that PICK1 moves transiently to synapses following
EGTA-AM) was adjusted to elicit calcium levels (as measured by
NMDAR activation, even at very early time points (1 – 2 min), we
fluo-3-AM fluorescence) comparable to those achieved by
do not see any evidence for this redistribution. Another possibility
activation of NMDARs (compare C). Control cells not
is that NMDAR activation triggers increased AMPAR endocytosis
loaded with caged calcium were exposed to the same stimuli to test
through AP2/dynamin-mediated mechanisms (
for nonspecific effects of photolytic damage. These controls
Lee et al., 2002), and PICK1 clusters by binding to these newly
showed no elevations in basal calcium levels (Five
internalized receptors. In fact, it has been demonstrated that
minutes following a ‘‘long'' stimulation (15 flashes) to generate
NMDAR activation increases the binding of PICK1 and GluR2
higher ‘‘NMDA/low Mg2+-like'' calcium responses, immunocyto-
(Interestingly, we find that PICK1 clustering
chemical labeling of PICK1 in neurons demonstrated a large
occurs under conditions that block GluR2 endocytosis. This
decrease in dendritic PICK1 levels (D,
suggests that AMPAR endocytosis is triggered in parallel with,
control levels, n = 8). In contrast, a shorter stimulus (7 flashes)
or as a result of, the recruitment of PICK1 to endosomes. In any of
generating ‘‘NMDA-like'' calcium responses resulted in an
these cases, our data demonstrating the localization of PICK1 away
increase in the net clustering of PICK1 (D, +65 T 9%
from synaptic sites support a model in which the primary role of
K.G. Sossa et al. / Mol. Cell. Neurosci. 31 (2006) 574 – 585
PICK1, under these signaling conditions, lies away from the
neurons (and because GluR2 is
synapse, and likely plays a role in maintaining AMPA receptor
dominant in the regulated endocytosis of AMPARs (
retention at endosomes.
al., 2004), it is not completely surprising that over the short
We find that PICK1 preferentially colocalizes with activated
time course of our experiments they behave similarly. Previous
rab5 over inactive rab5. Activated rab5 increases basal endocytosis
experiments that have demonstrated subtype-specific changes in
and promotes membrane fusion during endocytosis (
hippocampal neurons in response to elevated PICK1 levels
al., 1994). PICK1 clustering could reflect an association of PICK1
analyzed receptor expression at significantly later time points
with AMPARs, internalized by rab5 overexpression (
following manipulations (i.e. following 22 h of PICK1 over-
2005). If so, this may indicate that internalized AMPARs cluster
expression; Thus, the subtype-specific
PICK1 in the absence of activation of any other signaling
differences in expression may represent either delayed, com-
pathways. However, as we observe accumulation of PICK1 in
pensatory, or homeostatic changes that develop in response to
endosomes in the absence of GluR2 endocytosis, PICK1 may be
initial reductions in surface GluR2 levels. Consistent with this,
recruited to early endosomes independent of AMPAR expression in
we found that following a more prolonged reduction of PICK1
those vesicles. While the mechanism of this targeting remains to be
levels with siRNA treatment, there is a much greater effect on
determined, PICK1 has a BAR domain (a motif that enables the
GluR2 than GluR1.
binding of proteins to curved membranes), which in some proteins
The differential effects of NMDAR signaling on PICK1
has been found to interact with small GTPases that reside in
distribution appear to be linked to the absolute magnitude of the
trafficking endosomes (
calcium response elicited. Stimuli that induce large elevations in
PICK1 itself, through its PDZ domain, interacts with ARF1, a
calcium levels trigger decreases in dendritic PICK1 levels, while
GTPase that modulates vesicle trafficking at the Golgi (
stimuli that generate lesser calcium responses couple to increases
al., 2000). Further studies will be necessary to determine whether
in PICK1 clustering. Furthermore, direct manipulation of calcium
additional PICK1 interactions may serve to direct its localization
levels in neurons with flash photolysis demonstrates the impor-
under various signaling conditions.
tance of the magnitude of calcium response in mediating increases
We present a novel finding that a receptor-mediated signaling
and decreases in dendritic PICK1 levels. While the duration of the
pathway can initiate the unclustering of dendritic PICK1. Previous
calcium response may also be a factor in determining the
studies have reported that activation of NMDARs coupled to
redistribution of PICK1, the fact that a one-min exposure to
AMPAR endocytosis results in an increase in the binding of PICK1
NMDA was sufficient to drive the bidirectional changes in PICK1
and GluR2. In contrast, we find that strong NMDAR activation
clustering suggests that the size of the response plays a greater role.
reduces PICK1 labeling in dendrites and mediates the unbinding of
Additionally, we find that with strong activation of NMDARs, an
PICK1 and GluR2. This suggests that PICK1 unclustering might
intracellular calcium release component becomes important for
serve to initiate changes in the trafficking of AMPARs. In fact, we
effects on PICK1 distribution. Calcium-induced calcium release
find that the loss of PICK1 is accompanied by an increase in
(CICR) could simply provide a source of calcium necessary to
detectable surface AMPARs. That this change is dependent on
generate a sufficient magnitude of response, or may rather
PICK1 was confirmed by the observation that knockdown of PICK1
represent a spatially localized calcium signal that selectively
induces an increase in surface GluR2 that occludes any further
couples to PICK1 unclustering. Interestingly, direct activation of
NMDAR-mediated changes in surface AMPARs. PICK1 has been
calcium release has been reported to induce an increase in surface
proposed to contribute to the recycling of internalized AMPARs
AMPARs in a PICK1-dependent manner (
back to the membrane surface by mediating the dissociation of
However, our photolysis experiment, in which the magnitude
GluR2 from intracellular GRIP (
rather than the source of calcium is manipulated, suggests that the
2005). In this model, PICK1, together with activated PKC, is
magnitude of the response is the dominant factor. Postsynaptic
targeted to the site of GRIP bound AMPARs which then mediate
depolarization and direct or synaptic activation of NMDARs have
GluR2 Ser880 phophorylation and GRIP/GluR2 unbinding. How-
been shown to trigger potentiation or depression of synaptic
ever, our result, in which PICK1 is rapidly removed from endocytic
transmission in hippocampal pyramidal neurons, depending on the
vesicles, is more consistent with a separate mechanism by which a
extent of calcium entry through calcium channels (
population of PICK1-bound receptors is released for delivery to the
1992; Malgaroli and Tsien, 1992; Cummings et al., 1996; Lee et
membrane surface. The means by which this dissociation occurs is
al., 1998). The calcium-dependent redistribution of PICK1
unclear; however, studies have previously demonstrated the role of
provides one means by which differing levels of postsynaptic
the ATPase activity of NSF in mediating the unbinding of PICK1
activation could be coupled to forms of synaptic plasticity.
(enabling the synaptic insertion of AMPARs
We have investigated mechanisms that control surface AMPAR
(The activity of NSF itself may, therefore, be a
expression in response to glutamate receptor activation. We
point of regulation by NMDA receptors.
propose that activation of NMDARs differentially affects the
The heterologous overexpression of PICK1 in hippocampal
localization and clustering of dendritic PICK1. Through regulating
neurons results in a shift in the composition of synaptic
dendritic PICK1 expression, neurons can modulate surface
AMPARs; the surface levels of GluR2 being reduced while
AMPAR levels by mediating the numbers of internalized
levels of GluR1 elevated (In our
AMPARs. Future studies will determine the significance of PICK1
experiments, the surface GluR1 and GluR2 levels were both
clustering in the regulation of other proteins, given that PICK1
altered following glutamate receptor activation and PICK1
interacts with a number of pre- and postsynaptic proteins, including
redistribution, albeit the effects on GluR2 were slightly greater.
mGluR7a and EphBRs (While our results
However, due to differences in antibody characteristics, it is
have clear implications for the maintenance and regulation of
difficult to interpret these differences. Also, since GluR1 is
synaptic transmission, the further impact of PICK1 distribution on
thought to exist as a heteromer with GluR2 in hippocampal
overall neuronal physiology is likely.
K.G. Sossa et al. / Mol. Cell. Neurosci. 31 (2006) 574 – 585
Experimental methods
receptors were labeled with a fluorescently conjugated secondary antibody(1:300; 1 hr). Neurons were permeabilized in TBS/2%BSA/0.1% Triton-
Primary hippocampal cultures
X100 (30 min) and internalized AMPA receptors were labeled with adifferent secondary antibody (1:300; 1 h). Neurons were washed with 1
Cultured primary hippocampal neurons were prepared as described
TBS before mounting using Vectashield (Vector Laboratories, Burlingame,
previously (Hippocampi were isolated from P0 rats
(Sprague – Dawley) and the dentate gyrus was removed. Papain-treatedtissue was dissociated and plated on poly-l-lysine coated glass cover-
Assays for PICK1 Redistribution
slips. Cells were maintained in Neurobasal media (GIBCO) supple-
Cultures were treated with NMDA or NMDA/low Mg2+ (as described
mented with B27 (GIBCO) and glutamax (GIBCO, Invitrogen Corp.,
above) followed by fixation and permeabilization. PICK1 was labeled with
Grand Island, NY). All experiments were performed using cultures at 14
an N-terminal polyclonal antibody (1:1000, 1 h) and detected with a Cy3-
to 21 days in vitro.
conjugated secondary antibody.
Antibodies and reagents
Colocalization studies
Colocalization experiments employed various monoclonal markers of
Antibodies for immunocytochemistry included monoclonal anti-N-
sorting and trafficking machinery in conjunction with polyclonal PICK1 to
terminal GluR2 (Chemicon International, Temecula, CA), polyclonal
double label neurons. For early endosomal labeling, EEA1 (1:500; 1 h; BD
GluR1 (Calbiochem, La Jolla, CA), polyclonal PICK1 (Affinity Biore-
Transduction Laboratories, San Diego, CA) was used. For transferrin
agents, Golden, CO), EEA1 (Pharmingen, San Diego, CA), and
labeling, cultures were loaded with transferrin-tetramethylrhodamine (20
synaptophysin (Chemicon International, Temecula, CA). Secondary
Ag/ml; Molecular Probes, Inc., Eugene, OR) for 15 min before and during
antibodies (donkey anti-mouse or rabbit, Cy-3 or FITC-conjugated)
treatment with NMDA or NMDA/Low Mg2+. Following treatments,
were purchased from Jackson ImmunoResearch Laboratories (West
neurons were fixed, permeabilized, and labeled for PICK1 and above
markers as described. Positive colocalization was scored for discreteclusters of comparable size that were greater than 50% overlapping.
Analysis of EEA1 and transferrin experiments focused on dendrites 20 –100 Am away from the soma and was performed by investigators blind to
Glutamate receptors were activated using NMDA (50 AM; Tocris
Cookson, Inc., Ellisville, MO, with 10 AM CNQX also from Tocris) in thepresence of normal (1.3 mM) or of low (0.13 mM) magnesium for 1 to 2 min
as specified in each experiment. TTX was not included in most our
Immunolabeled neurons were imaged using a Hamamatsu Orca ER
experiments. A subset of experiments including TTX exhibited comparable
camera attached to an inverted Nikon fluorescent microscope with a 60
results. Neurons were washed in media and returned to 37-C for a total of 15
Plan Apo lens. Exposures were adjusted in each experiment to ensure that
min from the initiation of drug treatment. Cells were then fixed and processed
signals throughout the neuron were not saturated and fell within the linear
for immunocytochemistry. TPA (100 nM, Calbiochem) was applied to
range of the camera. Images were analyzed using Metamorph (Universal
neurons for 15 min prior to fixation and immunostaining. To determine the
Imaging, Inc., Downingtown, PA). Images were background subtracted and
calcium requirements of PICK1 redistribution, cells were incubated prior to
thresholded to include only signals about 2-fold greater than the diffuse
agonist application in BAPTA-AM (25 AM for 30 min; Sigma, St. Louis,
labeling in dendritic shafts. This process effectively highlighted the
MO) or cyclopiazonic acid (CPA; 25 AM for 15 min; Sigma, St. Louis, MO).
punctate labeling of PICK1 and surface AMPARs in dendrites. Integrated
To disrupt microtubule structure and elongation, cultures were incubated in
signal intensity values of PICK1 and surface AMPARs were determined for
colchicine (10 AM for 30 min, Tocris Cookson, Inc., Ellisville, MO) before
soma or dendrites, normalized to area, and were graphed as a percent
NMDA or NMDA/low Mg2+ treatment. In order to block endocytosis, a
change from labeling in untreated controls. For all experiments, except
hyperosmotic solution of sucrose (350 mM) was applied to neurons for 10
where noted, n values represent individual experiments in which 7 – 20 cells
min. For treatments where calcium concentrations were changed, artificial
are imaged in each.
cerebral spinal fluid (ACSF, contained (in mM): 119 NaCl, 26 NaHCO3, 10glucose, 2.5 KCl, 1 NaH
Mutant Rab5 overexpression
2PO4, 1.3 MgSO4, and 2.5 CaCl2) was used.
Nominally, zero calcium concentration was obtained by omitting the CaCl
Cultured neurons (11 – 14 DIV) were transfected with GFP-tagged
from ACSF. In calcium imaging and uncaging experiments, HEPES (10 mM)
Rab5 (Q79L = activated or S34N = inactivated) using lipofectamine
was used instead of NaHCO
2000 (Invitrogen Corp., Carlsbad, CA). Rab5 constructs were generously
3. Cultures were fixed and processed for
immunocytochemical analysis 15 min following initiation of agonist
donated by Brian P. Ceresa, Department of Cell Biology, University of
Oklahoma Health Sciences Center, Oklahoma City, OK (Ceresa, 2004). Neurons were incubated with the DNA-lipofectamine
2000 complex for 2 h before washing with conditioned media.
Following a 36-h incubation, PICK1 was immunocytochemically
Assay of surface receptors
detected. Colocalization analysis of PICK1 and GFP-Rab5 (mutants)
Mature (14 – 21 DIV) neuronal cultures were treated with NMDA/low
was done as previously described.
Mg2+ prior to live primary GluR2 antibody labeling (35 min at 4-C).
Following AMPAR labeling, neurons were fixed and blocked under
Coimmunoprecipitation and Western blot analysis
nonpermeabilizing conditions (TBS/4%BSA). Surface receptors were
Hippocampal slices (from 2 – 3 week old
immunocytochemically labeled with fluorescently conjugated secondary
rat pups were prepared and separated into 2 groups: control and treated with
NMDA/low Mg2+ (2 min followed by 13 min recovery period). Bothconditions were homogenized on ice in 25 mM HEPES-KOH (pH 7.8), 150
Assay of receptor endocytosis
mM NaCl, 3 mM MgCl2, 1 mM DTT plus ATPa˜S (Sigma-Aldrich Co., St.
Live cultures were labeled in conditioned growth media with GluR2 N-
Louis, MO) plus protease inhibitors (Complete Mini, EDTA-free, Roche
terminal antibody (10 Ag/ml) for 30 min. After antibody removal, cells were
Diagnostics, Mannheim, Germany). Following two cycles of homogeniza-
stimulated with glutamate receptor agonists. Fifteen minutes after initial
tion and centrifugation (1000 rpm, 5 min, 4-C), 1% TX-100 was added for
drug exposure, cells were fixed in paraformaldehyde (4%). Following
20 min while rocking at 4-C. Five micrograms of GluR2 antibody
blockade of nonspecific proteins in TBS, 2% BSA (30 min), surface AMPA
(Chemicon International, Temecula, CA) or nonspecific mouse IgG along
K.G. Sossa et al. / Mol. Cell. Neurosci. 31 (2006) 574 – 585
with 500 Ag of extract per condition was incubated overnight while rocking
Resources Program for Medical Schools and the NIH (NINDS)
at 4-C. After three washes with the above buffer, protein G agarose beads
(Sigma-Aldrich Co.) were added for 2 h to the extract/antibody mixture.
Bound proteins were separated from the beads by boiling for 5 min insample buffer. Immunoprecipitated and total lysate proteins were separatedon a 12.5% SDS/PAGE gel and PICK1 was detected by Western blotting
using PICK1 N-18 goat antibody (Santa Cruz Biotech, Santa Cruz, CA) andperoxidase-conjugated donkey anti-goat IgG (Jackson ImmunoResearch
Beattie, E.C., Carroll, R.C., et al., 2000. Regulation of AMPA receptor
Laboratories, West Grove, PA). Proteins were detected by enhanced
endocytosis by a signaling mechanism shared with LTD. Nat. Neurosci.
chemiluminescence (ECL, Amersham Biosciences, UK) and digitally
3 (12), 1291 – 1300.
imaged (Kodak Image Station 2000R; Kodak 1D v.3.6.1). Comparisons
Beretta, F., Sala, C., et al., 2005. NSF interaction is important for
were using the ratio of the mean intensity of the immunoprecipitated protein
direct insertion of GluR2 at synaptic sites. Mol. Cell. Neurosci. 28
to that of the total protein. Data are presented as a percent change from
(4), 650 – 660.
Braithwaite, S.P., Meyer, G., et al., 2000. Interactions between AMPA rece-
ptors and intracellular proteins. Neuropharmacology 39 (6), 919 – 930.
RNA knockdown of PICK1
Bredt, D.S., Nicoll, R.A., 2003. AMPA receptor trafficking at excitatory
Cultured neurons (9 – 12 DIV) were incubated with control scrambled
synapses. Neuron 40 (2), 361 – 379.
or PICK1 siRNA molecules (Invitrogen Corp., Carlsbad, CA) using
Brown, T.C., Tran, I.C., et al., 2005. NMDA receptor-dependent activation
lipofectamine 2000 for 2 h. After washing with conditioned media, infected
of the small GTPase Rab5 drives the removal of synaptic AMPA
neurons were incubated for 72 h. Subsequently, PICK1, GRIP (BD
receptors during hippocampal LTD. Neuron 45 (1), 81 – 94.
Transduction Laboratories), and surface GluR2-containing AMPARs were
Carroll, R.C., Beattie, E.C., et al., 1999. Dynamin-dependent endocytosis of
immunocytochemically labeled. Immunolabeling and treatments were
ionotropic glutamate receptors. Proc. Natl. Acad. Sci. U. S. A. 96 (24),
performed as previously described.
14112 – 14117.
Chung, H.J., Xia, J., et al., 2000. Phosphorylation of the AMPA receptor
subunit GluR2 differentially regulates its interaction with PDZ domain-
Regulated changes in calcium levels were detected in cultured neurons
containing proteins. J. Neurosci. 20 (19), 7258 – 7267.
loaded with fluo-3-AM (1 AM, Molecular Probes, Inc., Eugene, OR) for 20
Cummings, J.A., Mulkey, R.M., et al., 1996. Ca2+ signaling require-
min at 37-C in ACSF. Neurons were excited with a mercury arc lamp and
ments for long-term depression in the hippocampus. Neuron 16 (4),
imaged using a Nikon inverted fluorescent microscope objective (10).
825 – 833.
Changes in fluorescence were measured and recorded using Metamorph
Daw, M.I., Chittajallu, R., et al., 2000. PDZ proteins interacting with C-
(Universal Imaging, Inc., Downingtown, PA). Baseline fluorescence (Fo)
terminal GluR2/3 are involved in a PKC-dependent regulation of
was measured in artificial cerebral spinal fluid (ACSF) for 30 s before
AMPA receptors at hippocampal synapses. Neuron 28 (3), 873 – 886.
agonists were applied by perfusion (Warner Instruments, Hamden, CT).
Dinneen, J.L., Ceresa, B.P., 2004. Expression of dominant negative rab5 in
Effect on fluorescence by various treatments (F) was recorded for 10 min.
HeLa cells regulates endocytic trafficking distal from the plasma
Data were plotted as normalized changes in fluorescence from baseline
membrane. Exp. Cell Res. 294 (2), 509 – 522.
intensities (DF / Fo).
Ehlers, M.D., 2000. Reinsertion or degradation of AMPA receptors
determined by activity-dependent endocytic sorting. Neuron 28 (2),
511 – 525.
Two week old cultures were loaded with o-nitrophenyl-EGTA-AM
Gardner, S.M., Takamiya, K., et al., 2005. Calcium-permeable AMPA
(Molecular Probes, Inc., Eugene, OR) and Fluo-3-AM (Molecular Probes,
receptor plasticity is mediated by subunit-specific interactions with
Inc., Eugene, OR) for 45 min at 37-C in ACSF with HEPES. While
PICK1 and NSF. Neuron 45 (6), 903 – 915.
maintained in HEPES buffered ACSF, cells were given repeated dim
Habermann, B., 2004. The BAR-domain family of proteins: a case of
flashes to uncage calcium. Illumination was performed with a 100 W Hg
bending and binding? EMBO Rep. 5 (3), 250 – 255.
lamp filtered through a 300 – 400 nm band pass glass filter. Flashes (2 s in
Hansen, S.H., Sandvig, K., et al., 1993. Clathrin and HA2 adaptors: effects
duration regulated by computer controlled filter wheel (Sutter Instruments,
of potassium depletion, hypertonic medium, and cytosol acidification.
Novato, CA)) were repeated at 1 s intervals to induce cytosolic calcium
J. Cell Biol. 121 (1), 61 – 72.
rises similar to that observed in agonist treatment experiments. Fifteen
Henley, J.M., 2003. Proteins interactions implicated in AMPA receptor
flashes were required to induce a large calcium response while 7 were
trafficking: a clear destination and an improving route map. Neurosci.
sufficient to generate the smaller response. Cells were allowed to incubate
Res. 45 (3), 243 – 254.
at 37-C for 5 min following uncaging and then fixed and processed for
Iwakura, Y., Nagano, T., et al., 2001. N-methyl-d-aspartate-induced alpha-
immunolabeling of PICK1 or GluR2. Calcium responses were measured
amino-3-hydroxy-5-methyl-4-isoxazoleproprionic acid (AMPA) recep-
from multiple neurons in each experiment and are presented as averages.
tor down-regulation involves interaction of the carboxyl terminus of
Cells flashed in the absence of loaded caged calcium as well as cells loaded
GluR2/3 with Pick1. Ligand-binding studies using Sindbis vectors ca-
with cage that were not stimulated were also immunolabeled as
rrying AMPA receptor decoys. J. Biol. Chem. 276 (43), 40025 – 40032.
experimental controls. Controls showed no differences from labeling
Kim, C.H., Chung, H.J., 2001. Interaction of the AMPA receptor subunit
observed in naive cultures.
GluR2/3 with PDZ domains regulates hippocampal long-term depres-sion. Proc. Natl. Acad. Sci. U. S. A. 98 (20), 11725 – 11730.
Kullmann, D.M., Perkel, D.J., et al., 1992. Ca2+ entry via postsynaptic
voltage-sensitive Ca2+ channels can transiently potentiate excitatorysynaptic transmission in the hippocampus. Neuron 9 (6), 1175 – 1183.
Lee, H.K., Kameyama, K., et al., 1998. NMDA induces long-term synaptic
We thank Drs. D. Faber, K Kodakah, P. Castillo, and S. Zukin
depression and dephosphorylation of the GluR1 subunit of AMPA
for helpful comments in the preparation of this manuscript. Rab5
receptors in hippocampus. Neuron 21 (5), 1151 – 1162.
constructs were generously donated by Brian P. Ceresa, Depart-
Lee, S.H., Liu, L., et al., 2002. Clathrin adaptor AP2 and NSF interact with
ment of Cell Biology, University of Oklahoma Health Sciences
overlapping sites of GluR2 and play distinct roles in AMPA receptor
Center, Oklahoma City, OK. This work was supported by the
trafficking and hippocampal LTD. Neuron 36 (4), 661 – 674.
Whitehall Foundation, the Hughes Medical Institute-Research
Lee, S.H., Simonetta, A., et al., 2004. Subunit rules governing the sorting of
K.G. Sossa et al. / Mol. Cell. Neurosci. 31 (2006) 574 – 585
internalized AMPA receptors in hippocampal neurons. Neuron 43 (2),
Park, M., Penick, E.C., et al., 2004. Recycling endosomes supply AMPA
221 – 236.
receptors for LTP. Science 305 (5692), 1972 – 1975.
Lisman, J., 1989. A mechanism for the Hebb and the anti-Hebb processes
Perez, J.L., Khatri, L., et al., 2001. PICK1 targets activated protein kinase
underlying learning and memory. Proc. Natl. Acad. Sci. U. S. A. 86
Calpha to AMPA receptor clusters in spines of hippocampal neurons
(23), 9574 – 9578.
and reduces surface levels of the AMPA-type glutamate receptor
Lu, W., Ziff, E.B., 2005. PICK1 interacts with ABP/GRIP to regulate
subunit 2. J. Neurosci. 21 (15), 5417 – 5428.
AMPA receptor trafficking. Neuron 47 (3), 407 – 421.
Peter, B.J., Kent, H.M., et al., 2004. BAR domains as sensors of
Maher, B.J., Mackinnon II, R.L., et al., 2005. Activation of postsynaptic
membrane curvature: the amphiphysin BAR structure. Science 303
Ca(2+) stores modulates glutamate receptor cycling in hippocampal
(5657), 495 – 499.
neurons. J. Neurophysiol. 93 (1), 178 – 188.
Sheng, M., Lee, S.H., 2001. AMPA receptor trafficking and the control of
Malgaroli, A., Tsien, R.W., 1992. Glutamate-induced long-term potentia-
synaptic transmission. Cell 105 (7), 825 – 828.
tion of the frequency of miniature synaptic currents in cultured
Stenmark, H., Parton, R.G., et al., 1994. Inhibition of rab5 GTPase
hippocampal neurons. Nature 357 (6374), 134 – 139.
activity stimulates membrane fusion in endocytosis. EMBO J. 13 (6),
Malinow, R., Malenka, R.C., 2002. AMPA receptor trafficking and synaptic
1287 – 1296.
plasticity. Annu. Rev. Neurosci. 25, 103 – 126.
Takeya, R., Takeshige, K., et al., 2000. Interaction of the PDZ domain of
Matsuda, S., Mikawa, S., et al., 1999. Phosphorylation of serine-880 in
human PICK1 with class I ADP-ribosylation factors. Biochem.
GluR2 by protein kinase C prevents its C terminus from binding
Biophys. Res. Commun. 267 (1), 149 – 155.
with glutamate receptor-interacting protein. J. Neurochem. 73 (4),
Terashima, A., Cotton, L., et al., 2004. Regulation of synaptic strength and
1765 – 1768.
AMPA receptor subunit composition by PICK1. J. Neurosci. 24 (23),
Mulkey, R.M., Malenka, R.C., 1992. Mechanisms underlying induction of
5381 – 5390.
homosynaptic long-term depression in area CA1 of the hippocampus.
Wenthold, R.J., Petralia, R.S., et al., 1996. Evidence for multiple AMPA
Neuron 9 (5), 967 – 975.
receptor complexes in hippocampal CA1/CA2 neurons. J. Neurosci. 16
Murray, J.W., Wolkoff, A.W., 2003. Roles of the cytoskeleton and
(6), 1982 – 1989.
motor proteins in endocytic sorting. Adv. Drug Delivery Rev. 55 (11),
Xia, J., Zhang, X., et al., 1999. Clustering of AMPA receptors by the
1385 – 1403.
synaptic PDZ domain-containing protein PICK1. Neuron 22 (1),
Osten, P., Khatri, L, et al., 2000. Mutagenesis reveals a role for ABP/GRIP
179 – 187.
binding to GluR2 in synaptic surface accumulation of the AMPA
Zucker, R.S., 1999. Calcium- and activity-dependent synaptic plasticity.
receptor. Neuron 27 (2), 313 – 325.
Curr. Opin. Neurobiol. 9 (3), 305 – 313.
Source: http://ndm.dadatypo.net/files/resources/sossa-2006.pdf
Resident & Student Association rESidENt PrESidENt'S MESSagE 2012 Brings New Projects for aaEM/rSa Teresa M. Ross, MDAAEM/RSA President JM is a 35yo female with a history of recurrent calcium chloride, etomidate, fentanyl, furosemide, ketorolac, migraines. labetalol, ondansetron, phenytoin, prochlorperazine and rabies immunoglobulin.6 The majority of the medications are for iv use,
Upregulation of miR-142-3p in Peripheral BloodMononuclear Cells of Operationally Tolerant Patientswith a Renal Transplant Richard Danger,*† Annaïck Pallier,* Magali Giral,*†‡ Marc Martínez-Llordella,§Juan José Lozano, Nicolas Degauque,* Alberto Sanchez-Fueyo,§ Jean-Paul Soulillou,*†‡and Sophie Brouard*‡ *Institut National de la Santé Et de la Recherche Médicale UMR643 and Institut de Transplantation Urologie,Néphrologie, Nantes, France; †Université de Nantes, Nantes, France; ‡Centre Hospitalier Universitaire Hôtel-Dieu,Nantes, France; §Liver Unit, Hospital Clinic Barcelona, CIBEREHD, Barcelona, Spain; and Bioinformatics Platform,CIBEREHD, Barcelona, Spain