Pii: s0149-7634(98)00040-2
Neuroscience and Biobehavioral Reviews 23 (1999) 359±389
Neuropharmacology of brain-stimulation-evoked aggression
Allan Siegela,*, Thomas A.P. Roelingb, Thomas R. Gregga, Menno R. Krukc
aDepartment of Neurosciences, New Jersey Medical School, Newark, NJ 07103, USA
bDepartment of Anatomy and Embryology, Faculty of Medicine, University of Nijmegen, 6500 HB Nijmegen, The Netherlands
cMedical Pharmacology, LACDR, Sylvius Laboratory, University of Leiden, 2333 AL Leiden, The Netherlands
Received 1 May 1998; accepted 5 May 1998
Evidence is reviewed concerning the brain areas and neurotransmitters involved in aggressive behavior in the cat and rodent. In the cat,
two distinct neural circuits involving the hypothalamus and PAG subserve two different kinds of aggression: defensive rage and predatory
(quiet-biting) attack. The roles played by the neurotransmitters serotonin, GABA, glutamate, opioids, cholecystokinin, substance P, nore-
pinephrine, dopamine, and acetylcholine in the modulation and expression of aggression are discussed. For the rat, a single area, largely
coincident with the intermediate hypothalamic area, is crucial for the expression of attack; variations in the rat attack response in natural
settings are due largely to environmental variables. Experimental evidence emphasizing the roles of serotonin and GABA in modulating
hypothalamically evoked attack in the rat is discussed. It is concluded that signi®cant progress has been made concerning our knowledge of
the circuitry underlying the neural basis of aggression. Although new and important insights have been made concerning neurotransmitter
regulation of aggressive behavior, wide gaps in our knowledge remain. q 1999 Elsevier Science Ltd. All rights reserved.
Keywords: Aggression; Behavior; Attack; Limbic system; Rat; Cat; Neuroanatomy; Hypothalamus; PAG; Septal nuclei; Amygdala; Electrical stimulation;
Neuropharmacology; Receptors; Neurotransmitters; Serotonin; Acetylcholine; Dopamine; Norepinephrine; Glutamate; GABA; Neuropeptides; Opioids;
Cholecystokinin; Substance P; Agonist; Antagonist; Serenic; Psychotropic; Tranquilizer; Depressant; Electrical stimulation; Intracranial injection;
Over the past three decades, a number of investigators
In both cats and rats, aggression can be elicited by elec-
have electrically stimulated discrete brain areas in order to
trical stimulation of the hypothalamus and periaqueductal
evoke aggressive behavior in several species, mostly in cats
gray (PAG) of the midbrain, suggesting that aggression
and rats [12, 25, 34, 56, 75, 76, 98, 115, 119, 120]. Such
derives from similar neural substrates in both species
models of aggression possess three particular strengths.
[119, 120, 147, 206, 207]. However, the responses in rats
First, the responses closely resemble those that occur
differ greatly from those in cats, in terms of the form of
under natural conditions, particularly in the cat [126].
behavioral responses, speci®city of the neural substrate,
Second, the responses can be repeatedly elicited over
roles of speci®c neural structures (e.g. the PAG), involve-
many trials in a reliable manner, with stable latencies and
ment of neuroactive substances (e.g. serotonin and opioids),
thresholds. Third, because the responses are stable, an
and presumed function of the responses. Some of these
objective standard is provided by which changes in response
disparities may result from methodological limitations:
can be evaluated by statistical analyses. Thus, one can detect
since the cat has a larger brain and the dorsal surface of
subtle response changes that occur due to the infusion of
its skull is larger and sturdier than the rat's, complicated
drugs, either systemically or centrally, or due to other
techniques frequently used in catsÐsuch as infusing
physiological or environmental manipulations. This allows
drugs into a brain site and then electrically stimulating
investigators to characterize the neural circuits that produce
that site or another siteÐare only gradually becoming avail-
aggression. The present review describes the behavioral and
able for use in rats. By applying such techniques to the rat,
neural properties of these models of aggression and surveys
some of the apparent differences probably will disappear.
the literature concerning the roles of different putative
However, since the cat and rat occupy different ecological
neurotransmitter systems in the regulation of aggression.
nichesÐthe cat is a solitary, specialized carnivore, and the
rat is a colony-dwelling, opportunistic omnivoreÐit is to be
expected that different mechanisms have evolved to control
* Corresponding author. Tel.: 1 1-973-972-4471; fax: 1 1-973-972-
5059; e-mail:
[email protected].
aggression. By identifying the similarities and differences
0149-7634/99/$ - see front matter q 1999 Elsevier Science Ltd. All rights reserved.
A. Siegel et al. / Neuroscience and Biobehavioral Reviews 23 (1999) 359±389
between these species in which aggression subserves differ-
delivered to the attack site that can elicit the response, or
ent social and ecological functions, it is hoped that the prin-
alternatively as the current intensity eliciting the response in
ciples underlying the neural control of mammalian
50% of the trials; (2) response latency, de®ned as the time
aggressive behavior will be elucidated.
between onset of electrical stimulation and elicitation of the
response at a ®xed current level.
1. Behavioral aspects of brain-stimulation elicited attack
1.2. Using response patterns to categorize aggression
In ethology, both the releasing and directing stimuli and
the speci®c spatio-temporal pattern of a behavior are used to
1.1. Categorization and measurement of aggression
assess the motivational state'' of animals in natural
There is a semantic difference between cat and rat models
settings. It has been argued [112, 116] that since such
of aggression. In the cat, one can categorize stimulation-
motivational states'' are constructs derived from beha-
induced aggression as affective defense or predatory attack.
vioral observations, they may not have a simple relationship
In the rat, two types of motivational state in aggressive
to the activity of a speci®c brain mechanism. Therefore,
behavior, offense'' and defense'', have been postulated
there is a risk in classifying behaviors obtained by electrical
as categories [40, 112, 116, 218, 222]. These categories in
stimulation of a speci®c area into such motivational
rat and cat are based on different criteria. Affective defense
systems''. It is evident that the response pattern under
or defensive rage in the cat consists of threat postures, which
natural conditions will be affected by other controlling
may be followed by strikes upon provocation, similar to the
mechanisms in addition to the one activated by stimulation.
natural defensive behavior of a cat towards intruders in a
Therefore, it is essential to assess the factors (goal-object,
territory or towards other threats to itself or its offspring; this
stimulation intensity, behavior of the opponent) that deter-
behavior resembles both offense'' and defense'' in the
mine the spatio-temporal pattern of brain-stimulation-
rat. However, in the rat, threat behavior (lateral threat),
induced aggression. This issue is not without theoretical
which is mainly displayed by the resident in territorial ®ght-
impact. Many of the behavioral concepts currently used in
ing (except in the few cases in which it is exhibited by the
psychiatry and ethology are derived from the early in¯uen-
intruder instead), is labeled offensive'' behavior in
tial observations on brain-stimulation-evoked behavior in
contrast to the defensive'' attack behavior of a weaker
cats [96] and domestic fowl [225], made at a time when
intruder reacting to attacks by other rats [38±41]. Thus,
the disciplines of physiology and ethology were closer.
threat in defense of a territory in the cat has acquired the
1.3. Attack patterns in two feline models of aggression
label defensive'', whereas in the rat it has generally
elicited by electrical or chemical stimulation
acquired the label offensive''.
In the cat, the distinction between defense and predation
1.3.1. Quiet biting attack behavior
has been corroborated by neuroanatomical and pharmaco-
Quiet biting attack behavior, which is predatory in nature,
logical ®ndings [36, 55, 92, 93, 184, 197]. In contrast, in the
is typically characterized by stalking'' of a prey object
rat the distinction between offense'' and defense'' has
such as an anesthetized rat, followed by the biting of the
been made on the basis of a rather confusing mixture of
back of its neck. The attack begins after onset of stimulation
causation, function, form and the effect on the target of
and usually persists until stimulation is terminated. On occa-
the attack, not on the basis of neuroanatomical ®ndings;
sion, the cat may also strike the rat with its forepaw prior to
this distinction is not useful in understanding brain-stimula-
biting it [74, 227]. This behavior is remarkably similar to the
tion-induced aggression. For the ethological point of view,
response that occurs under natural conditions where stalking
see Ref. [218] for an excellent discussion of the problems
and killing of a rat in the open ®eld is readily observed
caused by such an approach. Recent evidence suggests that
[126]. Predatory attack is de®ned as occurring when the
in the rat, the neural substrate for stimulation-elicited
cat bites the target.
aggression is a single, multipurpose mechanism, releasing
attack whenever it is useful for survival, and that a single
1.3.2. Defensive rage behavior
area, the hypothalamic aggressive area (HAA), underlies
This form of aggressive behavior, originally described as
both offensive'' and defensive'' aggression, the differ-
affektive abwehr'' by Hess and Brugger [96], is character-
ences between them being due to differences in environmen-
ized by noticeable affective signs, including piloerection,
tal variables (see below). We will refrain from attributing
retraction of the ears, arching of the back, marked pupillary
observations of behavior to motivational'' systems impli-
dilatation, vocalization and unsheathing of the claws. The
cated by observations in natural settings, and we will retain
dependent variable often measured by experimenters is the
the straightforward nomenclature of the cat literature.
time between stimulation onset and onset of the audible
It should be noted that the two principal dependent vari-
portion of the hiss response. This response typically
ables used in these studies of aggression are: (1) response
involves mouth opening, baring of the teeth, an upward
threshold, de®ned either as the lowest electrical current
curling of the edges of the tongue, and then a hiss.
A. Siegel et al. / Neuroscience and Biobehavioral Reviews 23 (1999) 359±389
Fig. 1. (A) Distribution of sites within the preopticohypothalamus from which defensive rage (stippled area) and quiet biting attack (striped area) are most
frequently elicited by electrical stimulation. Number in upper left-hand corner of ®gure indicates the frontal plane of section. (B) Distribution of sites within the
PAG from which defensive rage and predatory attack can also be elicited by electrical or chemical stimulation. Note that, at the level of the hypothalamus,
predatory attack sites are situated lateral to those associated with defensive rage and, at the level of the PAG, defensive rage sites are situated dorsal to
predatory attack sites. Abbreviations: AH, anterior hypothalamus; F, fornix; IC, internal capsule; LH, lateral hypothalamus; MB, mammillary bodies; OT, optic
tract; RE, nucleus reuniens; VM, ventromedial nucleus. (From Ref. [201] with permission.)
Stimulation applied at medial hypothalamic sites from
pharmacology of narrowly circumscribed brain areas. In
which defensive rage can be elicited results in a dramatic
contrast, in rats, a more broad, general picture arises
activation of both sympatho-adrenal and cardiovascular
from studies involving either infusion of a chemical into
systems, producing marked increases in heart rate, blood
the brain, with subsequent observation of the behavioral
pressure, and peripheral epinephrine and norepinephrine
outcome, or peripheral administration of the substance,
levels [210].
followed by measurement of its effects on hypothalamic
Following its original description, this response was
attack. For example, recent work in stimulation-induced
given the misnomer sham rage'' because it was viewed
aggression in the cat focused upon the roles of opioid
as a pure motor act devoid of forebrain involvement [140].
and excitatory amino acid receptors within the PAG.
We now know that the forebrain is typically involved:
Such studies have not been carried out in the rat for two
defensive rage responses can be directed at speci®c moving
reasons. First, the cat is, at present, better suited than the
objects such as an awake rat, cat, or even the hand of an
rat for multiple brain manipulations. Second, in the rat the
experimenter. This response is a very real one, and it has
nonspeci®c opioid antagonist naloxone does not affect
ethological signi®cance: a nearly identical response can be
stimulation-induced attacks [112], and selective lesions of
evoked under natural conditions [126]. For example, this
the PAG only slightly and transiently affect hypothalamic
response may occur when a cat is intimidated by a threaten-
and territorial aggression [148]. Instead, in rats attention
ing stimulus, when a cat perceives that her kittens are endan-
has been paid to the role of serotonin receptors in the study
gered by another animal, or when a cat's territory is
of the effects of serenic'' drugs [112, 118, 157, 158, 219],
invaded. The typical sites from which predatory attack
as well as GABA, glutamate, and several other neurotrans-
and defensive rage can be elicited by electrical stimulation
mitters, as shown in Table 2.
are shown in Fig. 1.
1.4.1. Continuous diversity of hypothalamic attack patterns
1.4. Hypothalamic aggression in the rat: a different
Attacks elicited by hypothalamic stimulation in the rat
have been classi®ed into complete'' and incomplete''
attacks [108], and into affective'' and quiet attacks''
Different emphases are evident in the literature on
[161±163], jump attacks'', bite attacks'' [231], attack
feline and rodent aggression. In cats, emphasis has been
jumps'', and clinch ®ghts'' [117, 120, 219]. Such distinc-
placed on intensive study of the anatomy and behavioral
tions are necessary to describe the diversity of the attacks,
A. Siegel et al. / Neuroscience and Biobehavioral Reviews 23 (1999) 359±389
but the typology actually covers a continuum of forms, with
for survival. The form of the attack is determined by: (1) the
many intermediate forms and transitions between them.
intensity of stimulation current; (2) directing stimuli from
There is a general pattern in all attacks. Attack
the opponent; (3) the tactics the opponent adopts to avoid
patterns range in violence from mild biting of the neck
being attacked, e.g. hiding the attack-directing stimuli from
of an opponent through hard biting of the head and back,
the attacker. Curiously, the exact position of the electrode
through hard biting of the back accompanied by hind
within the HAA has relatively little effect on the form of the
paw kicks in the ¯ank, to clinch ®ghts and attack
attack [119, 120].
jumps [115, 117, 119]. The bite is always present and
First, at intensities below the threshold for attack, stimu-
the front paws may be placed at the opponent's neck or
lation makes the rat more self-directed and more sensitive to
back as a concomitant for biting. Subsequently, the hind
the activities of its partner. The partner reduces its physical
paws may come into action to kick the ¯ank, chest or
interactions with the stimulated rat. As stimulation intensity
belly of the adversary. Attack jumps arise when the
increases, the withdrawal of both rats from social interac-
opponent goes into an upright position, presumably to
tions also increases [90], but there are still no signs of
protect its back from being bitten. In attack jumps, the
aggression. At the approximate threshold intensity, the
attacker jumps from some distance toward the opponent,
mutual withdrawal from social interactions apparently
using its hind paws for takeoff and tail for balance and
fails and violent interactions take over, as bites directed at
support. Upon landing, the attacker tries to bite the head,
the back and neck of the partner are released. Further
while delivering a forceful blow with the hind paws
increases in the intensity of stimulation will shorten the
against the opponent's chest or belly. In clinch-®ghts,
latencies for attack and bias the attacks towards more
the rats often become locked in an on-top/on-back posi-
violent forms, accompanied by a tendency to use the hind
tion. Clinch-®ghts occur when one of the rats loses its
paws to kick at the body, in virtually all electrode place-
footing following an attack jump, or when an opponent
ments studied [114]. Increasing stimulation intensity seems
fails to assume an upright defensive position. From bite
to activate the attacker in cephalo-caudal order [113, 115,
attack to clinch ®ght and attack jump, there is a general
tendency to increase the arching of the back. The head
Second, strong directing stimuli on the head and rostral
and neck are the ®rst targets of attack followed by the
part of the back of the opponent are apparently essential
upper and lower back [115, 117, 147, 149]. Although a
components in shaping the form of the attack [117].
dominant rat could easily bite the exposed ventral surface
Third, the tactics of the opponent are important in deter-
of a submissive rat lying on its back, this surface is
mining the form of the attack, as mentioned above. Other
rarely bitten, even in a clinch ®ght [117]. Rats ®ghting
examples include the following. If the opponent freezes in a
in natural settings bite the same targets [38±41, 149].
crouched position, a bite accompanied by a kick of the hind
Hypothalamic attack can be directed against subordinate
paws often develops into a clinch ®ght. The treatment of
rats, dominant rats [108, 117], anesthetized rats, dead-and-
opponents with a high dose of morphine in order to alleviate
frozen rats [117] and mice [12, 105, 108, 161±163, 223,
pain and anxiety, a procedure which is now routine, causes
231]. Male rats also attack receptive or unreceptive females
them to stop emitting 22 kHz ultrasonic distress vocaliza-
[108, 117, 173]. Female rats attack other females as well as
tions and to stop adopting defensive upright positions.
males [114, 149]. In the absence of a rat-like object, attack
Consequently, attack-jumps are abolished (Fig. 2) and
does not occur. For example, rubber toys shaped like rats are
only straight attacks on the back occur. If the stimulation
not attacked [117]. Such ®ndings parallel the patterns
is strong, such attacks are followed by a clinch. Neither
observed in the cat, where stimulation-induced attack is
latencies to attack nor the threshold current intensity are
preferentially directed against live rats (as opposed to
changed. These observations show that while the active
dead or toy animals) [125].
participation of the opponent is not required to elicit an
Different strains of rats have different hypothalamic
attack, the opponent's defensive tactics affect the form of
attack patterns. For example, in the beige inbred CPB-
WEzob strain, bites on the head are the dominant pattern,
The hypothesis that these three factors allow for the
whereas in random-bred albino Wistar CPB-WE rats, the
observed variety in the form of ®ghts is supported by the
dominant pattern is a bite directed at the back, a pattern
fact that the intensity of the attack may be diminished by
which is absent from the inbred strain [115]. The attacks
decreasing stimulation intensity, treating the attacker with a
derive from the same anatomical area in both strains [115,
drug (e.g. l-propranolol; Kruk, unpublished results), or
treating the opponent with a drug such as morphine. In all
these cases the proportion of clinch ®ghts and jump-and-bite
1.4.2. Three factors determining the form of
attacks decreases considerably. These ®ndings also clarify
hypothalamically evoked aggression in the rat
why it is questionable to classify brain-stimulation-elicited
We argue that, upon stimulation of HAA in the rat, a
aggression as offensive'' or defensive'' on the basis of
multipurpose neural mechanism is activated that, under
super®cial similarities with behavior expressed in natural
natural conditions, subserves attack whenever it is required
A. Siegel et al. / Neuroscience and Biobehavioral Reviews 23 (1999) 359±389
Fig. 2. Changes in the attack pattern of six rats stimulated in the HAA, during attack threshold determinations lasting 15±20 trials, as a consequence of the i.p.
injection of 20 mg/kg morphine in 12 naive rats used as their opponents, compared with 12 control rats in a balanced design. Morphine injection 30 min before
the start of a threshold determination completely immobilizes and anesthetizes the opponents, but does not change the latency to attack or the attack threshold
in the untreated attacking rat (panels 1 and 2). However, morphinized opponents receive more hard-bites on their back (panel 3). Morphinized opponents
exhibit fewer clinch ®ghts than placebo-treated controls (panel 4). Moreover, morphinized opponents are not subject to jump-and-kick attacks, in contrast to
controls (not shown).
1.4.3. Behavioral concomitants of hypothalamic attack in
One concomitant response is self-stimulation. However,
the ability to elicit aggression cannot be predicted from the
In contrast to earlier observations [217], it is now known
rewarding or aversive properties of the stimulation. For
that stimulation of different hypothalamic areas yields
example, in one study, attacks were induced from 27 of
different behaviors [120±122]. Within the region from
51 electrodes aimed at sites in the HAA. In six of these
which attack can be elicited, other responses can be
sites the rats learned self-stimulation; in 11 sites they
obtained: piloerection, teeth chattering, quiet locomotion,
learned to switch stimulation off; in eight sites the rats
¯ight, escape, and self-stimulation. Some of these concomi-
learned both self-stimulation and the switch-off response,
tant responses are more prepotent than others. For example,
and in two sites they learned neither. From the 24 sites
piloerection is an inconsistent concomitant response, which
that did not produce attacks similar results were obtained
often disappears after a few stimulations. Each of these
[113]. A similar orthogonal relation between self-stimula-
behaviors has its own typical distribution in the hypothala-
tion and the elicitation of aggression was reported by Hern-
mus, sometimes overlapping with aggressive responses,
don et al. [95]. Because attack can be induced at sites that
sometimes as an independent response. Brie¯y, the anato-
produce self-stimulation, switch-off, and escape, as well as
mical distribution of teeth chattering sites closely matches
at sites that produce neither [113], it is likely that hypotha-
that of attack sites, whereas ¯ight and locomotion sites may
lamic attack in the rat does not derive from speci®c hedonic,
be located rostrally and caudally far beyond the region
aversive or ¯ight-inducing properties of stimulation.
where aggression can be elicited [120±123]. Teeth chatter-
When observing hypothalamic attacks in rats, one is
ing and piloerection are more prevalent in the area medial to
tempted to use concomitant behaviors accompanying attack
the fornix, whereas attack proper is more prevalent in the
as criteria to classify the attacks into motivational categories
subfornical area, giving way to social grooming in the direc-
such as predatory'', fear-induced'', irritation-
tion of the lateral hypothalamus. These ®ndings suggest that
induced'', offensive'', defensive'' or territorial''
in cat and rat one ®nds a similar diminution of affectivity''
aggression [151]. However, since the neural substrates for
when moving from medial to lateral through the hypotha-
several different neural systems are near to, or overlapping,
lamic area for aggression [121]. Piloerection is also a
one another within the hypothalamus, stimulation may acti-
component of the defensive attack pattern obtained from
vate several systems and thus produce a mixed'' combina-
the medial hypothalamus in the cat.
tion of responses. Therefore, in the rat it can be unclear
A. Siegel et al. / Neuroscience and Biobehavioral Reviews 23 (1999) 359±389
whether the responses elicited by stimulation arise from one
attack in the rat is the expression of a general-purpose attack
neural system or overlapping systems [172]. It is possible
mechanism used in all kinds of settings, regardless of their
that several of these concomitant responses form the overall
motivational'' nature. In natural settings, attacks probably
characteristic pattern of hypothalamically elicited attack
are released when modulatory brain mechanisms (e.g.
behavior. Therefore, one cannot use concomitant responses
medial amygdala, the prefrontal cortex, the septum or
to classify hypothalamic attack into motivational categories.
PAG) are suppressed. Indeed, stimulation of HAA appar-
However, such responses can serve as useful controls for
ently overrides or bypasses those inhibitory mechanisms
behavioral speci®city of drug effects [112, 118, 158, 219].
[112, 200, 201]. The mechanism that releases attack
presumably is not con®ned to the hypothalamus, but
1.4.4. Hypothalamic attack and natural'' aggression
includes such other regions as the PAG, medial amygdala,
Rat aggression has been studied extensively in experi-
lateral septum, and the ventral output pathways descending
mental paradigms where brain stimulation was not
caudally to the brain stem in the cat [201, 206] and rat [173,
employed. The attacks which occur in naturalistic para-
175] in different degrees depending on the setting in which
digms (such as in maternal aggression, offensive or defen-
aggressive responses are functionally required.
sive territorial aggression, mouse-killing, and in the
cornered rat'' paradigm) and in arti®cial paradigms
(such as shock-induced ®ghting) all share similarities with
2. Central pathways mediating attack behavior
hypothalamic attack. For example, the attack patterns and
In attempting to understand the neural substrates of
targets are similar. However, these attacks also differ from
aggression, two concepts and some de®nitions should ®rst
hypothalamic attack. Shock-induced ®ghting, for example,
be noted. First, a crucial distinction must be made between
is a purely defensive, re¯exive response to a painful stimu-
two classes of neural structures. The ®rst class of structures
lus [38±41]. It resembles the defensive response of an
produces the expression of an attack response when stimu-
attacked opponent in the upright position, but lacks the
lated. These structures, and the pathways arising from them,
purposeful, active approach and goal-directedness of
mediate, or carry the neural signals necessary for, the motor
hypothalamic attack. For a further comparison of hypotha-
and autonomic aspects of aggression. Sites within these
lamic aggression to aggression in other settings see Ref.
structures are termed, for example, defensive rage sites''
[112]. Such comparisons have led to the conclusion that
or predatory attack sites''. The second class comprises
hypothalamic attack in the rat is the consummatory
limbic structures whose stimulation does not produce the
response, or end-point behavior of the agonistic repertoire
expression of an attack response; rather, stimulation of
and, as such, constitutes a category in its own right. It
these structures increases or decreases thresholds
re¯ects the behavioral expression of a brain mechanism
( suppresses'' or facilitates'' the behavior respectively)
that is activated when control over the behavior of another
for responses elicited by concomitant stimulation of an
organism is lost, and agonistic strategies such as threats,
attack site. In other words, these structures modulate, or
displays and warnings are insuf®cient to reinstate control.
mediate the modulatory effects on'' aggression, and are
Under such conditions, inhibition over an attack±release
termed modulatory sites''. For example, medial amyg-
mechanism that can be activated from the hypothalamus is
daloid facilitation of PAG-elicited defensive rage'' is short-
overridden and a well-directed attack results. According to
hand for a decrease in the threshold for defensive rage
this concept, it is no surprise that the attack patterns
behavior elicited by electrical stimulation of the PAG,
observed during hypothalamic stimulation can also be
which occurs as a result of electrical stimulation of the
observed in territorial settings and resident±intruder para-
medial amygdala''. Second, while in the cat, the pathways
digms, in maternal aggression and in the cornered rat para-
mediating predatory attack and defensive rage are clearly
digm. Studies of feline hypothalamic attack addressing the
separate, this particular separation has not yet been found in
details of effector mechanisms, modulatory mechanisms,
the rat, although ongoing research is beginning to map out
and the speci®c sensory and endocrine changes involved,
the efferent pathways from the hypothalamic area where
support this view of the function of hypothalamic attack in
grooming can be elicited (hypothalamic grooming area;
natural settings [22, 73, 76, 206, 210, 211].
HGA) [176] and ¯ight-producing sites [173], as well as
It is not likely that hypothalamic stimulation brings the rat
HAA [173, 175]. The ®ndings of these studies generally
into a motivational state of aggression'' in the classical
parallel the ®ndings in the cat. The following discussion is
sense of the word [116, 128]. The effects of hypothalamic
based on work in the cat, referring to work in the rat when
stimulation are quick to appear, and they disappear quickly
after stimulation is terminated; also, lateral threat'' is
absent from these attacks. Some of the attacked targets
2.1. Central pathways mediating attack behavior in the cat
differ greatly from the targets attacked in natural agonistic
settings. Stimulation seems to elicit unplanned ®ghting
In the cat, the efferents from the hypothalamus and neigh-
which is out of context from the functional point of view.
boring regions of the midbrain PAG which subserve aggres-
These behavioral observations suggest that hypothalamic
sion were initially examined by Chi and Flynn [56, 57] and
A. Siegel et al. / Neuroscience and Biobehavioral Reviews 23 (1999) 359±389
the ventral half of the PAG, and the ventral and lateral
tegmental ®elds of the midbrain and pons (see Fig. 3).
The monosynaptic projection to the trigeminal motor
nucleus may activate jaw-opening and -closing mechanisms
for the biting component of the attack response. The projec-
tion to the locus coeruleus may serve two functions. First,
this nucleus projects to the intermediolateral column of the
spinal cord [58, 153]; this projection would thus complete a
disynaptic pathway for activation of the sympathetic
nervous system, which is integral to the process of both
predatory attack and defensive rage. Second, the locus coer-
uleus contains the largest pool of noradrenergic ®bers that
are distributed to the forebrain. Since the noradrenergic
system facilitates defensive rage [26, 27], it is likely that
these ascending ®bers provide a positive'' feedback
mechanism to the hypothalamus and limbic system. This
pathway could serve as a substrate for prolongation of the
attack response. Interestingly, central noradrenergic facili-
tation has also been proposed for aggression in the rat
(reviewed in Ref. [91]). In a parallel fashion, a similar func-
tion may be served by ascending ®bers associated with the
perifornical hypothalamus that supply modulatory struc-
tures such as the septal area, bed nucleus of the stria termi-
nalis (BNST) and preoptic region [49, 186, 208]. The septal
area is also involved in rat aggression [175].
Because the majority of efferents from predatory attack
sites in the ventral PAG and pontine tegmentum project to
the lateral hypothalamus [184], it is likely that these ®bers
may complete an additional positive feedback loop.
Descending projections from PAG predatory attack sites
pass for short distances to the lateral tegmental region
from which attack can be elicited [34] and to the pontine
Fig. 3. Diagram indicating the principal ascending and descending projec-
tions of the perifornical lateral hypothalamus associated with quiet biting
raphe complex, a region which, when stimulated, suppresses
predatory attack behavior. Note the projections to the PAG, tegmental
predatory attack [187]. The notion that the projection to the
®elds, locus coeruleus and motor nucleus of cranial nerve V. (From Ref.
raphe is associated with suppression of attack receives indir-
[201] with permission.)
ect support from the observation that parachlorophenylala-
nine (PCPA), which blocks the rate-limiting enzyme in the
later by others [75±77, 184]. The methods employed in
biosynthesis of serotonin, facilitates the occurrence of this
these studies included traditional tract-tracing methods
response [133]. Thus, it would appear that the ventral PAG
such as the Fink±Heimer method, tritiated amino acid auto-
modulates the activity of the perifornical hypothalamus,
radiography, and 14C-2-deoxyglucose autoradiography, as
where the primary integration for this response takes place.
reviewed elsewhere [201, 206].
2.1.2. Pathways mediating defensive rage behavior
2.1.1. Pathways mediating predatory attack behavior
The primary pathways associated with defensive rage
Quiet biting attack sites are located along the rostro-
arise from the medial hypothalamus [76, 77] and dorsal
caudal extent of the lateral hypothalamus, but the most
PAG [184]. Remarkably, ®bers arising from defensive
sensitive region is the perifornical region [201, 206]. In
rage sites in or near the ventromedial hypothalamus pri-
the brainstem, attack sites are located in the ventral aspect
marily pass rostrally, terminating principally upon neurons
of the PAG [184], ventral tegmentum [25] and as far caud-
within the medial anterior hypothalamus±preoptic zone, an
ally as the lateral tegmental ®elds of the pons [34]. The most
area from which this response could also be elicited. Inputs
important cells which give rise to ascending and descending
to this zone include axons from limbic regions such as the
®ber systems mediating the expression of predatory attack
amygdala, septal area and BNST [48, 49, 211]. The
are situated in the perifornical lateral hypothalamus.
descending ®bers which innervate the PAG mostly originate
Descending ®bers from the lateral hypothalamus project to
from this zone (see Fig. 4). This pathway from medial
nuclei that appear to be essential for predatory attack,
hypothalamus to dorsal PAG constitutes the principal path-
including the trigeminal motor nucleus, the locus coeruleus,
way for defensive rage in the cat. These ®ndings provided
A. Siegel et al. / Neuroscience and Biobehavioral Reviews 23 (1999) 359±389
Fig. 4. Diagram indicating the principal ascending and descending projections of the medial hypothalamus associated with defensive rage behavior. Note that
®bers mainly ascend from the ventromedial hypothalamus to the anteromedial hypothalamus and preoptic region and that ®bers that supply the PAG arise
principally from the anteromedial hypothalamus. (From Ref. [206] with permission.)
the basis for further studies, described below, concerning
behavior [201, 202, 205]. Because little is known about
the role of catecholamines and excitatory amino acids in
the transmitters involved, we will only brie¯y discuss
this pathway.
pathways from the amygdala.
In contrast to the predatory attack system, the majority of
Several important modulatory pathways issue from the
®bers arising from PAG defensive rage sites pass caudally,
amygdala. The most well-documented pathway, the stria
to the locus coeruleus, sensory and motor nuclei of the
terminalis, arises from the medial nucleus, medial aspects
trigeminal complex, and neighboring regions of the
of the basal complex, and the area in and around the cortical
midbrain and pontine tegmentum. The projections to the
nucleus. These ®bers arise from modulatory regions which,
locus coeruleus may serve the same two functions as
when stimulated, facilitate defensive rage and suppress
noted above for the predatory attack system. Other path-
predatory attack [42, 48, 70, 211]. The primary target of
ways governing autonomic regulation may include ®bers
these ®bers is the medial hypothalamus, in which they
from the caudal PAG which appear to descend to autonomic
terminate over a wide area, extending from the anterior-
nuclei of the lower brainstem [24]. The efferents to the
preoptic zone to the posterior aspect of the ventromedial
trigeminal complex may mediate the vocalization that
nucleus [110, 211, 228]. In contrast, ®bers which arise
normally accompanies defensive rage. Similar to the preda-
from the central nucleus, lateral aspects of the basal
tory attack system, some of the ®bers arising from the dorsal
complex, and the lateral nucleus are distributed to the
PAG project rostrally to portions of the medial hypothala-
BNST and, via the ventral amygdalofugal pathway, through
mus that produce defensive rage, and may complete a simi-
the medial forebrain bundle to the lateral hypothalamus,
lar positive feedback loop, allowing for the response to be
PAG, and autonomic nuclei of the caudal medulla. It has
prolonged. However, it should be noted that the PAG
been shown that the cells of origin of these ®bers are asso-
contains sites which, when stimulated, can facilitate or
ciated with suppression of defensive rage and facilitation of
suppress defensive rage or predatory attack elicited by
predatory attack [42, 48, 70]. Thus, strikingly, some cell
hypothalamic stimulation [165, 229]. The pathways from
groups in the amygdala facilitate predatory attack but
the PAG for predatory attack and defensive rage are
suppress defensive rage and other cell groups do the oppo-
summarized in Fig. 5.
site. It is likely that the outputs of the amygdala to the
medial hypothalamus and PAG constitute direct routes by
2.1.3. Limbic pathways that modulate predatory attack and
which the amygdala modulates aggressive reactions, and the
output to the BNST is an indirect route. Evidence in support
Limbic structures give rise to pathways that synapse upon
of this indirect route is that: (1) the BNST projects directly
hypothalamic or PAG cells and modulate aggressive
to the medial hypothalamus and PAG [97, 228]; (2) BNST
A. Siegel et al. / Neuroscience and Biobehavioral Reviews 23 (1999) 359±389
Fig. 5. Diagrams indicating the principal efferent projections from PAG sites associated with defensive rage (left side) and predatory attack (right side). Note
that ®bers associated with defensive rage arising from the PAG are distributed rostrally to the medial preopticohypothalamus from where this response can also
be elicited and caudally to the locus coeruleus, tegmental ®elds and trigeminal complex. Also note that the distribution from predatory attack sites is more
limited in which a primary projection ascends and synapses in the posterior lateral hypothalamus where this response can also be elicited. Descending
projections supply the central tegmental ®elds and median raphe. (From Ref. [206] with permission.)
stimulation results in modulation of both defensive rage and
with the systematic distribution studies by Kruk and cowor-
predatory attack [186]; (3) infusion of an opioid agonist into
kers [114, 119, 120], taking into consideration the dimen-
the BNST results in suppression of defensive rage [51]. The
sions of electrodes and stimulation techniques used, it seems
nucleus accumbens modulates defensive rage elicited from
clear that all these responses have been induced in a subfor-
the medial hypothalamus [52].
nical area (Fig. 6). It is likely that all these responses were
obtained from the same neural system within that area.
2.2. Neural substrates of aggressive behavior in the rat
HAA extends laterally from the arcuate nucleus and
medial aspect of the ventromedial nucleus to the ventral
2.2.1. Relationship of anatomical loci to hypothalamic
aspect of the lateral hypothalamus. It extends rostrally
attack responses and structure of the hypothalamic attack
from the lateral edge of the ventromedial nucleus towards
the frontal pole of the ventromedial nucleus and anterior
The ®rst studies in rat, conducted with limited numbers of
hypothalamic nucleus [113, 114, 119, 120]. HAA is
implanted electrodes, suggested that different types of
approximately the same in male and female rats [114,
aggression could be induced from different hypothalamic
119] as well as in different strains of rats [115, 120]. Lesions
areas. In contrast to these reports [12, 105, 108, 161±163,
of HAA reduce aggression evoked by an intruder in a terri-
223, 231], it was later found that the whole region of the
torial setting [8, 154, 159]. Marginal sites within HAA yield
HAA basically yields the same set of aggressive responses,
less intense responses. These studies suggest that a speci®c
and that the variations in response can be accounted for by
hypothalamic mechanism is involved in the control of
the intensity of stimulation, posture of the opponent, and the
aggression, although the sites producing aggression are
simultaneous activation of concomitant responses [114,
not limited to a single nuclear group. See Figs. 6 and 7.
116, 118, 120±123]. If one compares the regions of the
Following a novel classi®cation of hypothalamic areas
hypothalamus where other investigators [12, 105, 108,
based on cytoarchitectonic criteria [81, 82], it is now
161±163, 173, 223, 231] elicited aggressive responses
recognized that HAA almost completely coincides with
A. Siegel et al. / Neuroscience and Biobehavioral Reviews 23 (1999) 359±389
Fig. 6. Attack-inducing sites in the hypothalamus of the rat plotted in the atlas of Geeraedts et al. [81, 82]. Adapted from Ref. [175]. The attack area largely
coincides with the intermediate hypothalamic area, and the ventro-lateral pole of the ventromedial nucleus of the hypothalamus. Abbreviations: AHA, anterior
hypothalamic area; ci, capsula interna; DHA, dorsal hypothalamic area; DMH, dorsomedial hypothalamic nucleus; fx, fornix; LHN, lateral hypothalamic
nucleus; LPOA, lateral preoptic area; mt, mammillothalamic tract; ot, optic tract; PFX, perifornical nucleus; PVH, paraventricular hypothalamic nucleus; sm,
stria medullaris; VMH, ventromedial hypothalamic nucleus; ZI, zona incerta.
the intermediate hypothalamic area (IHA). IHA is about
setting [148]. This differs from the cat, in which such lesions
0.5 mm3 in size, and contains about 17 £ 103 neurons and
block aggression elicited by stimulation of the stria termi-
about 150 £ 106 synaptic contacts. About half of these
nalis [72]. In the rat, though HAA efferents clearly differ
contacts are symmetrical contacts, the other half being
from the efferents of the HGA, which is located in the para-
asymmetrical. The synapse-to-neuron ratio is about 9 £
ventricular nucleus and adjacent dorsomedial hypothala-
103, whereas there are only 170 axo-somatic contacts per
mus, they may not be speci®c for attack behavior, since
soma. IHA may be homologous to the anterior hypothala-
lesions of the PAG do not signi®cantly affect hypothalamic
mus in the cat. The projection from the lateral septal area to
attack or hypothalamic self-grooming [148, 220]. The
the HAA consists of small unmyelinated varicose ®bers
effects of hypothalamic stimulation are possibly mediated
forming axo-dendritic asymmetrical contacts in the IHA
by the ventro-caudal projections of HAA (see also [9]).
[1]. We assume that hypothalamic aggressive responses
Stimulation of HAA activates ascending projections
are elicited by the overriding of local tonic inhibition,
terminating in and projecting through the medial preoptic
since local infusion of the GABAA antagonist bicuculline
area [173, 175] where, occasionally, attack has been
induces similar attacks [174]. Similar results were reported
induced in the proximity of the ventral supraoptic commis-
for the GABA antagonist picrotoxin [9], and for the combi-
sure. This commissure, which may connect to neurons in the
nation of a glutamate agonist and bicuculline [91].
IHA/HAA or the adjacent anterior hypothalamus, has been
suggested as a key element in the attack-relevant mechan-
2.2.2. Efferent pathways involved in hypothalamic attack in
isms in the hypothalamus [173]. Moreover, Adams [9] has
shown that the GABA antagonist picrotoxin elicits aggres-
In the rat, as in the cat, the hypothalamic attack area
sive behavior when injected into the area where electrical
projects extensively to the PAG. In rat, in the regions of
stimulation elicits attack, but also in the adjacent frontal
the PAG which receive projections from HAA, attack simi-
area, ventrally in the anterior hypothalamus.
lar to hypothalamic attack can be elicited, although not as
In the cat, clear-cut anatomical boundaries are present for
easily as from HAA [147], and the responses are sometimes
different aggressive responses. In the rat, the apparent
accompanied by severe motor disturbances. However,
absence of such boundaries has complicated the task of
selective destruction of the entire PAG only slightly and
tracing the connections of the system to more caudal regions
transiently reduces attack elicited by hypothalamic stimula-
of the brainstem, where a similar form of attack can be
tion and aggression provoked by an intruder in a territorial
induced by electrical stimulation [147, 226]. However, the
A. Siegel et al. / Neuroscience and Biobehavioral Reviews 23 (1999) 359±389
projections of the IHA have now been studied in detail using
that the majority of the projections from these two areas
the Phaseolus vulgaris leucoagglutinin (PHA-L; see Fig. 7)
have nothing to do with the speci®c behavioral conse-
[175]. It now seems likely that HAA projects to many areas
quences of stimulation, or that the behavioral speci®city is
that affect aggression [226]: its projections pass through
due to subtle differences in projections within the target
several of the structures relevant for aggression in the cat,
structures. There are indications for the latter explanation.
such as the ventral tegmental area (VTA), raphe magnus,
Fig. 7 illustrates how HGA and HAA efferents have differ-
PAG, locus coeruleus, the A5 region of the brainstem
ent preferences for speci®c structures. As expected, HGA,
tegmentum, and the nucleus tractus solitarius, and are
but not HAA, projects clearly to the arcuate nucleus and
covered with varicosities or boutons-en-passant, which
median eminence. Moreover, HGA projects much more
may release neurotransmitter into these structures. These
extensively to the ventral tegmental area, raphe magnus,
projections presumably subserve similar functions as
locus coeruleus and dorsal motor nucleus of the vagus and
suggested for the cat (i.e. autonomic or endocrine integra-
nucleus tractus solitarius. HGA projects to ventrolateral
tion, or feedback loops). For a discussion of the involvement
PAG, whereas HAA projects to dorsal and dorsolateral
of endocrine mechanisms and hypothalamic behavioral
PAG. Since aggressive behavior is associated with high
regulation see Ref. [66].
arousal, and grooming is associated with the absence of
arousal, it is interesting to note that the parts of the PAG
2.2.3. Speci®city of the projections from HAA/IHA
efferent to HAA are associated with blood pressure
PHA-L tracing studies by Roeling and coworkers [175,
increases whereas parts efferent to HGA are associated
176] make it possible to get an impression of the behavioral
with decreases. HAA projects preferentially to the adjacent
speci®city of HAA and HGA efferents. These studies
ventromedial nucleus of the hypothalamus and to the para-
revealed eight different streams of thin unmyelinated vari-
taenial and mediodorsal thalamic nuclei; HGA projects
cose efferents from each of these areas. The streams from
preferentially to the medial preoptic area and anteroventral
HAA split and end in at least 26 different areas, projecting
parts of the periventricular nucleus. The most pronounced
frontally as far as the nucleus accumbens and the lateral
differences between HGA and HAA are found in the
septum, dorsally as far as the lateral habenular nucleus
projections to the lateral septal nucleus: HGA projects
and caudally as far as the nucleus of the solitary tract and
almost exclusively to the ventral part of this nucleus,
ventrolateral medulla. The projections from HGA are simi-
whereas the HAA projects almost exclusively to the dorso-
lar. Fibers also emerge from HGA in eight unmyelinated
lateral portion of the intermediate part [175, 176]. The septal
streams, covered with varicosities, follow paths very similar
projections of the HAA are of interest because the lateral
to those from HAA, and terminate in at least 23 areas. At
septum sends projections to the HAA, suggesting that the
least 14 brain areas receive similar projections from both
rage that is a well-known consequence of the interruption of
HGA and HAA (Table 1). These similarities suggest either
septal±hypothalamic connections [10, 11, 13±16] may be
Fig. 7. Schematic representation of the speci®c projections of the hypothalamic attack area (HAA), as contrasted with the projections speci®c to the
hypothalamic grooming area (HGA). For details on the 16 areas to which both HAA and HGA project (not shown here) see Refs. [175, 176]. Abbreviations:
Arc, arcuate nucleus; CGD, dorsal part of the PAG; CGld, dorsal portion of the lateral part of the PAG; CGlv, ventral portion of the lateral part of the PAG;
DMV, dorsal motor nucleus of the vagal nerve; LC, locus coeruleus; LSIdl, dorsolateral portion of the intermediate part of the lateral septal nucleus; LSV,
ventral part of the lateral septal nucleus; MD, mediodorsal thalamic nucleus; ME, median eminence; MnPO, median preoptic nucleus; NTS, nucleus of the
solitary tract; PeAV, anteroventral part of the periventricular nucleus; Pt, parataenial thalamic nucleus; RMg, raphe magnus; VMH, ventromedial hypothalamic
nucleus; VTA, ventral tegmental area.
A. Siegel et al. / Neuroscience and Biobehavioral Reviews 23 (1999) 359±389
according to this model, four visual areas (the lateral super-
Brain areas receiving projections from both the hypothalamic grooming
ior colliculus, the lateral pretectal area, and the dorsal and
area (HGA) and the hypothalamic aggression area (HAA), according to
ventral lateral geniculate nuclei) are preferentially activated
PHA-L injections (adapted from Refs. [175, 176]). Only areas that received
projections in all rats injected at attack sites and all rats injected at groom-
during HAA stimulation, but the PAG is not.
ing sites are listed
Some support for this model is given by the facts that: (1)
hypothalamic aggression has, on occasion, been elicited by
stimulation of the region anterior and ventral to the HAA,
Anterior commissure
proximal to the optic tract; (2) the HAA extends from the
Bed nucleus stria terminalis
Central tegmental ®eld
IHA into the ventrolateral part of the VMH [119, 123].
However, only the VMH, zona incerta and cuneiform area
Lateral hypothalamic area
have been shown to be connected directly to the HAA, and
Lateral habenular nucleus
only the ventrolateral part of VMH is considered as part of
Lateral preoptic area
the aggression-relevant network in the study by Roeling and
Lateral septal nucleus, intermediate part
Lateral septal nucleus, ventral part
coworkers. Roberts' study suggests that the peripeduncular
Mesencephalic PAG
nucleus is most speci®cally associated with the HAA, and
Medial amygdaloid nucleus
the septal area has no place in Roberts' proposed model
Medial preoptic area
[173]. Roeling and coworkers suggest that the most speci®c
Paraventricular thalamic nucleus
output pathway for the HAA is a speci®c part of the lateral
Substantia innominata, pars subcommisuralis
Substantia innominata, pars sublenticularis
septum, and the peripeduncular nucleus is absent from the
projections studied by them. It should be noted that, in the
cat, a pathway from the lateral hypothalamic-predatory
due to interruption of a feedback loop [10, 11, 13, 14, 16,
attack region to the lateral septal area has been identi®ed
43]. Moreover, HAA projections form peculiar pericellular
[75]; these authors interpreted this pathway as a feedback
baskets around septal cells that could interact with the sero-
tonergic baskets around septal cells [80]. Thus one can spec-
Four methodological reasons could explain the con¯ict-
ulate that the effects of serotonin agonists on hypothalamic
ing conclusions derived from these rat studies. First, the
attack [112, 113] and on aggression induced by other means
brain activity in anesthetized animals which are continu-
[155, 157, 158] are due to interference with a HAA±septal
ously stimulated for long durations, at suprathreshold inten-
feedback loop.
sities, may differ from the activity induced during the
The fact that HAA projects to an area does not prove that
milder, short stimulations used to elicit attacks in awake
such a projection mediates hypothalamic attack. Clues
animals. Second, even though the electrodes are in the
about the involvement of speci®c areas could be derived
same area as in other studies (i.e. in the IHA), larger areas
from the study of the patterns of activation caused by stimu-
may have been activated. Third, stimulation may also have
lation of IHA/HAA. However, an extensive study by
had antidromic effects; therefore, deoxyglucose methods
Roberts and Nagel, utilizing deoxyglucose uptake as a meta-
may reveal projections to and from the HAA, whereas
bolic marker during stimulation of sites in HAA [173], is
PHA-L reveals only the latter. Fourth, the emphasis on the
hard to reconcile with the PHA-L projection studies. In this
predominance of contralateral effects in the deoxyglucose
study, hypothalamic sites that produced upward ¯ight beha-
study may be due to the statistical method used to demon-
vior were used as controls for speci®city of effects. Deoxy-
strate behavioral speci®city by contrasting hypothalamic
glucose uptake in 62 sites was quanti®ed. Deoxyglucose
¯ight against hypothalamic attack. However, the raw data
labeling was more intense on the side of the brain ipsilateral
in that study clearly show that the ipsilateral effects are at
to stimulation in both attacking rats and controls. Thirty-two
least as prominent as the contralateral effects. The conclu-
brain areas were signi®cantly more activated in attacking
sion can only be that many pathways may be responsible for
rats than in controls. Curiously, there were more differences
stimulation-induced aggression, and that additional studies
from the controls at contralateral sites (28 differences) than
and novel methods (e.g. c-Fos) are required to unravel the
ipsilateral sites (21 differences). In contrast, in the PHA-L
studies, contralateral projections from the HAA/IHA were
not much in evidence [175]. Based upon the deoxyglucose
2.2.4. Functional relationships of other limbic structures to
data and data from the general anatomical literature, but not
hypothalamic attack in the rat
from the PHA-L studies, a model was proposed by Roberts.
The HAA overlaps with the projection areas of the medial
According to this model, the efferents for aggression run
amygdala to the medial hypothalamus [113, 114, 119, 120,
from the HAA, via the ventromedial nucleus of the hypotha-
130]. Infusion of arginine-vasopressin (AVP) into the
lamus (VMH), to the ipsilateral and contralateral ventral
medial amygdala increases territorial aggression and
supraoptic commissure to continue to the ventral zona
partially counteracts the gradual decrease in territorial ®ght-
incerta, the subparafascicular nucleus, the peripeduncular
ing caused by castration [109]. It is known that the expres-
nucleus and the cuneiform area in the brain stem. Also
sion of endogenous central vasopressin is dependent on
A. Siegel et al. / Neuroscience and Biobehavioral Reviews 23 (1999) 359±389
testosterone. Therefore, it is notable that castration causes
region. Third, with the microinjection technique, drug
increases in hypothalamic attack thresholds that can be
concentrations may exceed concentrations of the amine or
reversed by testosterone [33]. Ovariectomy does not affect
peptide that normally acts at these receptor populations; for
thresholds [114]. Infusion of the serotonergic drug, quipa-
this reason, it is possible that systemic and intracerebral
zine, into the medial amygdala reduces natural mouse kill-
microinjections of a compound may yield different results.
ing [166]. In addition, the medial amygdala in the rat and cat
Fourth, if the volume of injection is too large, the drug may
seems to have a role in the learning of social signals and the
diffuse through the brain to affect areas other than the target
signi®cance of defeat [3, 109, 130, 224]. As noted above,
area, as may have been the case for Ref. [99].
connections of the amygdala with the hypothalamus may
In the rat, much work has examined the involvement of
control hypothalamic attack release mechanisms with
neurotransmitters in aggression. However, since most of
respect to these aspects of aggression.
these studies have not used brain stimulation to elicit
Stimulation of the deeper orbital layers of the prefrontal
aggression, they fall outside the scope of this review. See
cortex inhibits attacks elicited from the hypothalamus in rats
the review by Miczek et al. [142] for an account of this
[65]. This parallels the ®ndings in the cat, where stimulation
of the lateral or medial prefrontal cortex blocked predatory
attack elicited from the lateral hypothalamus [203, 204].
3.1. Evidence for the presence of a cholinergic mechanism
The pathway mediating this effect may be a multisynaptic
projection from the prefrontal cortex to the lateral hypotha-
lamus, via the mediodorsal and midline nuclei of the thala-
3.1.1. Systemic injections
Several early studies demonstrated that systemic injec-
tions of muscarinic agonists could facilitate defensive rage
in the cat [83, 124, 234]. Berntson and Leibowitz [37]
3. The neuropharmacology of brain-stimulation-induced
extended these ®ndings by showing that injections of the
aggressive behavior
muscarinic agonist, arecoline, could induce biting attack,
which, in turn, was blocked by pretreatment with the
Studies of neurotransmitter involvement in stimulation-
muscarinic antagonists atropine or scopolamine. A later
induced aggression have employed two approaches. In the
study [35] showed that pretreatment with nicotine can
®rst approach, an agonist and/or antagonist for the putative
suppress naturally evoked or arecoline-induced predatory
transmitter is injected systemically and the effect of the
attack and defensive rage. Similar results were also
injection upon the threshold or latency is measured over
observed by Katz and Thomas [100] who noted that the
time. This approach can provide an overall assessment of
threshold for hypothalamically elicited predatory attack
whether the transmitter is involved in aggression. In addi-
was elevated following scopolamine administration.
tion, it has the advantage of constituting the clinical route of
However, Baxter [30] noted that atropine or scopolamine
administration of drugs such as carbamazepine. The de®-
had little effect upon hypothalamically elicited defensive
ciency of the approach is that it does not reveal the locus of
rage, other than to produce ataxia. Interestingly, scopola-
action of the drug. In addition, with the use of this approach,
mine does not affect thresholds for hypothalamic attack in
one must determine whether the effects of drug administra-
rats; perhaps the stimulation overrides or bypasses local
tion are speci®c to the process under investigation. In the
cholinergic blockade [112, 118].
second approach, the agonist or antagonist is microinjected
into a speci®c region within the brain, usually at an attack
3.1.2. Intracerebral injections
site, and the effects of drug infusion are identi®ed. It should
Further support for the notion that muscarinic receptors
be noted that the use of cannula-electrodes allows investi-
are involved in the expression of aggression was gained
gators to electrically stimulate an area and to infuse a drug
from several studies. Beleslin and Samardzic [31] observed
or retrograde tracer into the same area. This approach can
hissing and growling following intraventricular injections of
provide clues about the presence or absence of a putative
muscarine chloride, which could be blocked by atropine or
transmitter at a given synaptic region and its possible role in
scopolamine. Such effects have also been reported when
aggression, with four caveats. First, it is entirely possible
carbachol was placed into the medial hypothalamus [45,
that a receptor is present at a synapse, while the neurotrans-
46, 99, 177, 178, 221] or PAG [29, 94]. In an extensive
mitter usually associated with that receptor is not present. In
mapping study, Allikmets [17] injected acetylcholine into
such a case, the drug would act upon receptors at the
defensive rage sites and observed similar behavioral effects;
synapse, resulting in a change of behavior, and this would
these sites were primarily localized to the periventricular
lead one to the false conclusion that the transmitter at that
hypothalamus and PAG. Defensive rage can also be
synapse has been identi®ed. Second, this approach can lead
observed following cholinergic stimulation of the septal
to a ®shing expedition'' in which many different drugs are
area [94] or dorsomedial amygdala [18], areas usually
tested without a suitable rationale, unless there is a sound
considered to be modulatory. However, these areas have
rationale that the transmitter is present at the synaptic
low thresholds for epileptiform activity, and stimulation of
A. Siegel et al. / Neuroscience and Biobehavioral Reviews 23 (1999) 359±389
them produces attack behavior only at current levels which
is the only drug that facilitates hypothalamically elicited
produce seizures [200, 201]. Thus, the responses observed
attack [118].
by these authors may have been secondary to seizure activ-
Other indirect evidence in support of a serotonin mechan-
ity induced by chemical stimulation. Somewhat surprising
ism is that acute treatment with the tricyclic antidepressants
results were obtained by Kono and coworkers [106, 107]
chlorimipramine and imiprimine (which may be more effec-
who administered intrahypothalamic injections of acetyl-
tive against catecholamines) suppresses predatory attack in
choline or carbachol. They noted that low doses of these
the cat [68, 69]. These drugs are believed to inhibit prefer-
drugs did not alter spontaneous behaviors, but suppressed
entially the uptake of amines in serotonergic neurons when
electrically elicited defensive rage. In contrast, higher doses
administered acutely [53]. In addition, if the animal is
elicited defensive rage. Further, choline or physostigmine
pretreated with PCPA, chlorimipramine does not suppress
elevated attack thresholds, whereas nicotine was ineffective;
attack, suggesting that endogenous levels of serotonin are
these inhibitory effects were antagonized by atropine but not
required for the antidepressant's inhibitory effects. Further-
curare, further supporting the notion that muscarinic
more, when the animals were treated with the serotonin
mechanisms are involved. They also observed that the
precursor 5-hydroxytryptophan (5-HT), the suppressive
effects of microinjections of acetylcholine into the medial
effects of chlorimipramine were restored. It is of interest
hypothalamus were blocked when lesions were placed into
to note that acute imipramine administration does not
the stria terminalis, which led them to conclude that this
suppress defensive rage [68] and, in fact, even facilitates
pathway is cholinergic [107].
this response [137, 164]; this may be due to an elevation
Regarding predatory attack, one study reported that
of norepinephrine levels (see discussion below). Chronic
carbachol microinjections into the ventral tegmentum of
administration of tricyclics might have different effects on
cats produced defensive rage followed by directed killing
feline aggression. It is possible that since tricyclics, like the
of a mouse [99]. The fact that both types of aggression
selective serotonin reuptake inhibitor paroxetine, elevate
followed the injections suggests that they activated a wide
levels of 5-HT in the synaptic cleft when administered
area containing separate regions for each form of attack.
acutely, they, like paroxetine, also lead to a down-regulation
These studies indicate that cholinergic drugs act through
of 5-HT2 receptors when given chronically. Since acute
muscarinic receptors in the medial hypothalamus and PAG
treatment of rats with antidepressants results in a decrease
to modulate defensive rage and (possibly) predatory attack.
in aggression, whereas chronic treatment results in an
However, a more convincing experiment to show that acet-
increase, an effect possibly due to down-regulation of 5-
ylcholine is involved in aggression would require that
HT2 receptors [146], perhaps chronic treatment of cats
microinjection of a cholinergic antagonist into a synaptic
with tricyclic antidepressants would enhance predatory
region critical for the expression of attack inhibits that
attack and/or defensive rage.
response when elicited either under natural conditions or
by stimulation of another attack site.
3.2.2. Intracerebral injections
3.2. Evidence for serotonergic involvement in attack
In two separate studies, Golebiewski and Romaniuk [86]
behavior in the cat
and Romaniuk et al. [179] demonstrated that serotonin,
injected into hypothalamic sites from which carbachol can
3.2.1. Systemic injections
elicit affective defense, inhibits the attack response. Conver-
A serotonergic mechanism may be involved in aggres-
sely, when methysergide, a serotonin antagonist, is injected
sion, as suggested by the consistent effects of systemically
into such sites, the attack response is enhanced. Moreover,
injected PCPA. For example, in one study PCPA induced
when 5,6-dihydroxytryptamine (5,6-DHT), which selec-
both hypersexuality and predation [71]. Furthermore,
tively destroys serotonin neurons, is microinjected into the
several studies showed that PCPA facilitates (i.e. shortens
dorsal raphe, there is a decrease in brain serotonin coupled
the latency and lowers the threshold for) hypothalamically
with an enhancement in carbachol-induced defensive rage.
elicited predatory attack [100, 133]. One site where seroto-
Thus, it is reasonable to conclude that serotonin normally
nin probably acts to modulate this response is the trigeminal
serves to suppress defensive rage by acting, at least in part,
motor nucleus, which is needed for biting to occur. Support
upon neurons in the medial hypothalamus.
for this view was provided by MacDonnell and Fessock
The raphe system projects to the forebrain, brainstem
[132] who demonstrated that evoked potentials in the
tegmentum, limbic system and even the trigeminal motor
trigeminal motor nucleus elicited by stimulation of parts
nucleus. A recent set of experiments tested the effects of
of the basal ganglia from which jaw-opening could also
microinjections of selective 5-HT compounds into the PAG
be elicited were enhanced by PCPA treatment. These effects
upon defensive rage elicited from the medial hypothalamus
may not be limited to the cat: PCPA reduces natural preda-
[188]. Defensive rage was suppressed following microinjec-
tion time of the grasshopper mouse upon crickets [141].
tions of the 5-HT1A receptor agonist, 8-OHDPAT; pretreat-
Regarding defensive rage behavior, systemic administration
ment of the PAG with microinjections of the 5-HT1A
of PCPA facilitates this response [133]; and in the rat, PCPA
antagonist p-MPPI blocked this effect. Facilitation of
A. Siegel et al. / Neuroscience and Biobehavioral Reviews 23 (1999) 359±389
defensive rage followed microinjections of the 5-HT2/1C
of hypothalamic attack in the rat by a limited class of sero-
agonist, (1) DOI hydrochloride [188].
tonergic drugs, acting perhaps through 5-HT1B receptors,
However, these studies did not fully identify (1) the sites
seems to parallel the overall suppressive effects of this
where serotonin affects neurons associated with attack, (2)
neurotransmitter upon aggressive reactions reported above
the actions of the respective 5-HT subtypes upon aggres-
sion, or (3) the cellular mechanisms of action of each of the
The behavioral selectivity of the effects of serotonergic
drugs was demonstrated by the fact that other behavioral
consequences obtained from the same electrodes in the
same animals were hardly affected by these drugs. Con-
3.2.3. Effects of serotonergic agents upon hypothalamic
versely, stimulation-induced locomotion was facilitated by
attack in the rat
scopolamine and amphetamine at doses that did not affect
In an initial set of experiments, PCPA was shown to
hypothalamic attack elicited from the same sites. The phar-
decrease 5-HT levels and facilitate hypothalamic attack
macologic pro®le of stimulation-induced teeth chattering
[118]. Similarly, infusion of 5,7-DHT, into the hypothala-
and escape resembles the pro®le of attack.
mus resulted in similar facilitation of offensive'' aggres-
sion in territorial ®ghting [222]. For a review of the
involvement of serotonin and other transmitters in aggres-
sive behavior, see Ref. [143].
In a series of studies, the effects of serenic''
compounds (which have high af®nities for 5-HT recep-
The overall results obtained in cat and rat indicate that
tors [158]) upon hypothalamic attack thresholds were
serotonin mechanisms suppress aggression. Moreover, for
determined using an up-and-down procedure (variation
the rat, the combined results of studies involving lesions,
of the Method of Limits) [112, 114, 117, 118, 219,
infusions of selective neurotoxins into the hypothalamus [8,
230]. Drugs and doses were chosen which severely affect
154, 160, 222], or application of selective anti-aggressive
territorial or maternal aggression. Whenever possible,
drugs acting through 5-HT receptors, strongly suggest that
concomitant responses such as teeth chattering, locomo-
the hypothalamic attack release mechanism is involved in
tion and escape elicited from the same electrodes were
aggression in natural settings.
used as controls for behavioral speci®city. Details on
The following line of evidence supports this conclusion.
procedures can be found in Refs. [118, 219]. Table 2
Fluprazine and TFMPP are prototypes of the so-called
summarizes the effects of drugs on thresholds, obtained
serenics'' which have high af®nities for 5-HT1 receptors
by different groups in different strains of rats. The effects
[158], and have been shown to inhibit hypothalamic attack
are expressed as percentage increase with respect to vehi-
selectively in both sexes and in different strains (CPB-
cle control. All drugs were tested in adult males, except
WEzob rats, albino random bred CPB-WI Wistar rats and
alcohol which was tested in adult females. All drugs
Tryon Maze Dull rats) [112, 114, 118, 157, 158, 219].
have been shown to inhibit aggression in natural settings
Serenics also reduce natural'' aggression provoked by
such as territorial or maternal aggression, and usually at
the presence of an intruder endangering offspring or terri-
lower doses than used here. Only a few drugs strongly
tory [155±158, 160]. Behavioral sequence analysis of the
increased thresholds. The phenyl-piperazines quipazine,
effects of an early prototype of the serenics [155] in a terri-
serenic'' compounds ¯uprazine and its metabolite
torial setting reveals that serenics do indeed inhibit the most
TFMPP, but also dl-propranolol, selectively elevated
violent parts of the agonistic pattern such as ®ghting and
thresholds and also displayed steep parallel dose±effect
biting. The rest of the agonistic pattern remains relatively
curves, suggesting a common mechanism of action.
unaffected by these drugs. Thus, drugs that inhibit hypotha-
Fluvoxamine and oxazepam weakly affected thresholds.
lamic attack also inhibit attack in natural settings. In
In contrast, dl-amphetamine, scopolamine, 8-OH-DPAT
contrast, many drugs that do not inhibit hypothalamic attack
(a selective 5-HT1A agonist), mianserine (a 5-HT2 antago-
do inhibit aggressive behavior in a territorial setting [160].
nist) and chlordiazepoxide had no effect.
However, drugs such as scopolamine and amphetamine
The inhibitory effects of propranonol could be due to its
seem to achieve such effects by reducing aggressive
af®nity for central 5-HT receptors [88, 144, 145] or to adre-
postures due to the induction of stereotypy and locomotion
nergic blocking properties. The drug has a stereospeci®c
effect on territorial aggression [233] and hypothalamic
It has been suggested that the anti-aggressive properties
attack (Kruk, unpublished results). Fluprazine and propra-
of the serenics are due to anxiety-promoting properties [101,
nolol facilitate social cooperation between rats in the same
102]. Since the potent anxiolytic oxazepam slightly inhibits
way [32]. Interestingly, propranolol and TFMPP have simi-
hypothalamic attack, such a conclusion may not be justi®ed.
lar discriminative stimulus properties [85].
Anxiogenics, such as inverse benzodiazepine agonists, have
Strikingly, many drugs that affect aggression in natural
just begun to be studied [see section 3.9 for further
settings do not affect hypothalamic attack. Thus, modulation
A. Siegel et al. / Neuroscience and Biobehavioral Reviews 23 (1999) 359±389
Pharmacological pro®le of hypothalamic attack for systemically administered drugs in rats. Drugs that suppress hypothalamic attack increase the threshold
current required to induce attack. The increases are expressed as a percentage of the vehicle condition. Only the effects at the maximum dose are given.
Adapted from Refs. [112, 118, 158, 219]. Notice that PCPA (parachlorophenylalanine) facilitates hypothalamic attack, and hypothalamic attacks are relatively
insensitive to many drugs that do affect other forms of aggressive behavior, and that, apart from the dopaminergic antagonist haloperidol, drugs displaying
serotonergic agonism are among the most effective drugs
Increase from control (%)
Dose range (mg/kg)
0.5±2.0 i.p.
0.25±1.0 i.p.
50 (at highest dose only)
250±2000 p.o.
0.5±2.0 p.o.
1.25±10 i.p.
100 single dose p.o.
50 single dose i.p.
0.05±0.2 s.c.
0.125±1.0 p.o.
1.25±10 i.p.
375 single dose i.p.
3.3. Dopaminergic involvement in stimulation-induced
D1 antagonist, SCH 23390. Thus, it was concluded that
attack behavior in the cat
dopaminergic facilitation of defensive rage is mediated
primarily by D2 receptors [212].
3.3.1. Systemic effects
Predatory attack is also facilitated via D2 receptors [190].
Dopaminergic mechanisms are thought to facilitate
Apomorphine administration facilitated the occurrence of
defensive rage and predatory attack. One indirect piece of
this response; and spiperone, but not SCH 23390 (a D1
evidence is that electrical stimulation of the ventral tegmen-
antagonist), elevated response thresholds when adminis-
tum or substantia nigra, the primary sources of dopaminer-
tered alone, and blocked the facilitatory effects of apomor-
gic innervation of the forebrain, facilitated defensive rage
phine when delivered before apomorphine. In conclusion, it
[63, 185]. Direct evidence was obtained by Maeda et al.
would appear that dopaminergic mechanisms facilitate
[136] and Maeda and Maki [135] who showed that thresh-
defensive rage and predatory attack, whereas serotonin inhi-
olds for hypothalamically elicited defensive rage decreased
bits these responses.
following systemic administration of the indirect and direct
dopamine agonists methamphetamine and apomorphine
3.3.2. Intracerebral injections
respectively. Maeda and coworkers also showed that halo-
Dopaminergic ®bers from the ventral tegmentum and
peridol, a non-selective dopamine antagonist, elevated the
adjoining regions of the far medial hypothalamus project
defensive rage threshold.
rostrally to the limbic forebrain and supply, among other
Another study replicated and extended these ®ndings.
regions, the medial anterior hypothalamus±preoptic zone, a
First, it was found that haloperidol injected alone elevated
region critical for the expression of defensive rage in both
thresholds, or, when injected before apomorphine, blocked
cat and rat. Accordingly, the model shown in Fig. 8
its facilitatory effects. Then, these authors found that the
proposes that catecholaminergic ®bers facilitate defensive
relatively selective D2 agonist, LY 171555 (Quinpirol),
rage, particularly at the level of the anterior medial hypotha-
but not the selective D1 agonist, SKF 38393, facilitated
lamus (although interactions at other levels along the
defensive rage. Moreover, the facilitatory effects of apomor-
limbic-midbrain axis may also exist).
phine or LY 171555 could be selectively blocked with
This hypothesis was directly tested in one study [213].
pretreatment with either haloperidol or the speci®c D2
Stimulating electrodes were implanted into the region of the
antagonist, spiperone, but not with the relatively selective
ventromedial hypothalamus and cannula-electrodes were
A. Siegel et al. / Neuroscience and Biobehavioral Reviews 23 (1999) 359±389
Fig. 8. Schematic diagram indicates the proposed circuitry involved in catecholaminergic facilitation of defensive rage behavior (elicited from the ventro-
medial hypothalamus). Note that an important site of interaction where catecholaminergic ®bers produced facilitation of this response is the anterior
hypothalamus. Abbreviations: AH, anterior hypothalamus; AUTO, autonomic cell groups of lower brainstem; DA, dopaminergic ®bers; NE, noradrenergic
®bers; PAG, midbrain periaqueductal gray; SOM, somatic cell groups of lower brainstem; VM, ventromedial nucleus.
implanted into the medial anterior hypothalamus±preoptic
induced escape, had no effect on hypothalamic aggression
zone. Dopaminergic compounds were microinjected into
defensive rage sites within the medial anterior hypothala-
In an early study [198], systemic administration of
mus±preoptic zone. In brief, microinjections of apomor-
amphetamine enhanced the facilitatory effects of reticular
phine or a relatively selective D2 agonist, LY 171555 (but
formation stimulation upon predatory attack elicited from
not a D1 agonist, SKF 38393) facilitated defensive rage (see
the hypothalamus. Marini et al. [138] later demonstrated
Fig. 9). Moreover, microinjections of either the non-selec-
that low doses of dl-amphetamine facilitated predatory
tive antagonist, haloperidol, or the D2 antagonist, sulpiride,
attack and higher doses inhibited it. When catecholamine
but not the D1 antagonist, SCH 23390, elevated response
levels in the brain were depleted following a-methylpara-
thresholds when injected alone into the anterior medial
tyrosine administration, a cat's approach toward a rat was
hypothalamus, and blocked the effects of apomorphine or
blocked but re¯exive biting remained intact [100]. In
LY 171555 when administered as a pretreatment. This result
contrast, after catecholamine levels were antagonized by
demonstrates that dopaminergic stimulation of the medial
the dopamine b-hydroxylase (noradrenergic synthesis)
anterior hypothalamus±preoptic zone facilitates defensive
blocker, disul®ram, hypothalamically elicited predatory
rage behavior via D2 receptors.
3.4. Noradrenergic involvement in stimulation-induced
Early experiments suggested that norepinephrine facili-
tates attack. However, the data were not entirely consistent.
One line of investigation [169±171] demonstrated that a
selective decrease in brain norepinephrine follows defensive
rage reactions produced by hypothalamic stimulation in the
cat. These authors further showed that pre-collicular lesions
produced recurrent defensive rage responses which were
accompanied by decreases in norepinephrine content in
the brainstem (in contrast to mid-collicular lesions, which
were not associated with either defensive rage responses or
changes in brainstem norepinephrine). The authors
concluded that a fall in norepinephrine content is speci®c
for defensive rage and further suggested that the central
release of norepinephrine triggers the attack response.
3.4.1. Systemic injections
Several investigators examined the effects of d-ampheta-
mine, which causes release and prevents the reuptake of
Fig. 9. Time course of the effects of microinjection of LY 171555 (50 and
catecholamines, upon predatory attack and defensive rage
250 ng/0.25 ml) or vehicle (0.1% ascorbic acid) into the medial preoptico±
in the cat. Amphetamine administration was reported to
anterior hypothalamic area upon ventromedial-hypothalamically elicited
facilitate defensive rage in one study [19], but in another
hissing. Each point represents the average change in hissing threshold
it had no effect upon this response when elicited from the
current expressed as a percentage relative to preinjection baseline thresh-
old. Arrow indicates time of injection. *p , 0.05 compared with vehicle.
medial hypothalamus [30]. In rats, systemic dl-ampheta-
Bars S.E.M.; n 5 for each treatment. (From Ref. [213] with
mine, at doses that affect locomotion and stimulation-
A. Siegel et al. / Neuroscience and Biobehavioral Reviews 23 (1999) 359±389
suppressed this response as the animals appeared lethargic
and placid. In another study, Romaniuk and Golebiewski
[180] microinjected norepinephrine into sites within the
medial hypothalamus of the cat where carbachol could elicit
defensive rage and failed to observe any changes in
In more recent experiments [26, 27], whose rationale was
virtually identical to that described above for Ref. [213], the
underlying hypothesis was that noradrenergic ®bers modu-
late neurons within the anterior medial hypothalamus.
Accordingly, infusion of noradrenergic compounds into
the hypothalamus should affect hypothalamically elicited
defensive rage. In brief, infusion of either norepinephrine
or the a-2 agonist, clonidine, into defensive rage sites within
the anterior medial hypothalamus, facilitated that response
when it was elicited from the ventromedial hypothalamus.
Moreover, pretreatment with the a-2 antagonist, yohimbine,
blocked the effects of both norepinephrine and clonidine. In
contrast, phenylephrine (a selective a-1 agonist), proprano-
lol (a b-blocking agent), terbutaline (a b-2 agonist), meto-
prololartrate (a b-1 antagonist) and butoxamine (a b-2
antagonist) had little or no effect on attack when micro-
injected alone or when used as a pretreatment for norepin-
ephrine. Therefore, it is reasonable to conclude that the
facilitating effects of norepinephrine upon defensive rage
Fig. 10. Proposed model of functional relationship between amygdala,
hypothalamus, and PAG with respect to the control of defensive rage beha-
are mediated, in part, by actions on a-2 receptors in the
vior and predatory attack behavior. Note that processes excite defensive
anterior medial hypothalamus. See Fig. 8.
rage behavior by driving neurons in either the medial hypothalamus (MH)
or midbrain periaqueductal gray (PAG). Because of the presence of an
3.5. The role of neuropeptides in the regulation of feline
inhibitory GABAergic neuron that projects from the medial to lateral
aggressive behavior
hypothalamus (LH), excitation of the mechanism for defensive rage will
inhibit the mechanism for predatory attack. Cholecystokinin, norepineph-
3.5.1. Opioid peptides
rine, dopamine, and serotonin inputs are not shown but are important
Opioid involvement in aggression is suggested by the
modulators of defensive rage and predatory attack. Other abbreviations:
AB, basal amygdaloid complex; AM, medial amygdaloid nucleus; CE,
following evidence. First, it has been suggested, at the clin-
central amygdaloid nucleus; AUTO, autonomic cell groups of lower brain-
ical level, that morphine reduces heightened levels of
stem; SOM, somatic cell groups of lower brainstem; EAA, excitatory amino
aggressiveness [103, 104, 232]. Second, in the cat, opioid
acids; ENK, enkephalin; SP, substance P; 1 , excitatory pathway; 2 ,
receptors and enkephalin-containing cells and axon termi-
inhibitory pathway.
nals are present in dense quantities in the PAG, central
attack was strongly inhibited [132]. While these studies
nucleus of the amygdala, BNST, and nucleus accumbens
suggest that predatory attack in the cat is facilitated by
[20, 87, 89, 150, 167, 191, 192, 206].
noradrenergic mechanisms, one study conducted in the rat
3.5.1.1. Systemic studies in the cat. Three ®ndings suggest
reported inhibition of hypothalamic attack after systemic
that opioids selectively suppress defensive rage. First,
injection of the b-blocker, propranolol [112].
systemic administration of naloxone lowered the threshold
for defensive rage elicited from hypothalamus or PAG, in a
3.4.2. Intracerebral injections
dose-dependent manner [50, 195]. Second, predatory attack
Torda [214] induced defensive-like aggression in rats
thresholds from hypothalamic sites were elevated by
with the aid of foot shock and then observed that infusion
naloxone administration [50], whereas thresholds for
of a cocktail of norepinephrine and dopamine into the
PAG-elicited contralateral circling were unaffected. Third,
medial hypothalamus facilitated this response. On the
®ghting behavior induced in cats by intraventricular
other hand, contrasting ®ndings were observed in another
injections of carbachol was suppressed by subsequent
study [84], where it was found that intraventricular infusions
intraventricular injections of morphine, suggesting that m
of dopamine increased shock-induced ®ghting, but norepi-
receptors may be important [111].
nephrine reduced ®ghting. With respect to the cat, Mark et
al. [139] induced defensive rage by placing lesions in the
3.5.1.2. Intracerebral injections in the cat. The BNST and
ventromedial hypothalamus. They observed that norepi-
possibly the nucleus accumbens may receive opioidergic
nephrine injections into the basolateral amygdala
inputs from the central nucleus of amygdala [216], and
A. Siegel et al. / Neuroscience and Biobehavioral Reviews 23 (1999) 359±389
Fig. 11. (a) Morphiceptin, in doses of 0.05±0.4 nmol, suppressed PAG-elicited defensive rage in a dose- and time-dependent manner; (b) pretreatment with
0.2 nmol of b-FNA 5 min prior to morphiceptin administration into the same sites blocked the suppressive effects of morphiceptin (0.05 nmol). However,
pretreatment with b-FNA at a dose level of 0.05 nmol did not block the suppressive effects of either dose of morphiceptin. Bars S.E.M. (From Ref. [191] with
enkephalinergic cell bodies and axon terminals are present
at these sites would prevent the enkephalins from suppres-
in the PAG [150].
sing the attack mechanism (see schematic diagram in
Therefore, in separate experiments, the nonspeci®c enke-
phalin agonist d-ala2-met5-enkephalinamide (DAME) was
There also exist PAG sites which facilitate and suppress
microinjected into sites within the BNST and nucleus
predatory attack behavior elicited from the lateral hypotha-
accumbens which modulated hypothalamically elicited
lamus. These modulatory sites are in¯uenced by naloxone,
defensive rage [51, 52]. At both of these nuclei, DAME
suggesting that the enkephalinergic mechanism regulates
elevated thresholds for modulation. Moreover, pretreatment
predatory attack at the level of the PAG [229].
of these sites with naloxone blocked the effects of DAME.
At least two questions were left unanswered by these
This suggests that hypothalamically elicited (or PAG-
studies. The ®rst concerns the relevant subtypes of enkepha-
elicited) defensive rage may be governed by an opioidergic
linergic receptors, and the second relates to whether enke-
input from the central nucleus, which, when activated,
phalinergic neurons situated outside the PAG project
suppresses defensive rage. In fact, amygdaloid inputs can
directly to defensive rage sites within the PAG. These two
modulate the activity of neurons in the BNST via an opioid
questions were addressed in three experiments. In the ®rst
mechanism [64].
experiment [194], DAME was infused into PAG defensive
Two studies demonstrated that defensive rage [165] and
rage sites and it was observed that thresholds increased.
predatory attack [229] are modulated within the PAG by an
Pretreatment with naloxone blocked the suppressive effects
opioidergic mechanism. In one study [165], defensive rage
of DAME, and DAME did not affect PAG-elicited circling
was elicited from the medial hypothalamus and cannula-
behavior. The conclusion was that enkephalinergic recep-
electrodes were placed into PAG sites which inhibited or
tors in the PAG mediate suppression of defensive rage. In
facilitated this response. The results indicated that infusion
the second experiment, Shaikh et al. [191] sought to identify
of naloxone into inhibitory, but not facilitatory, PAG sites
the receptor subtypes involved in the suppression of defen-
blocked the modulatory effects on hypothalamically elicited
sive rage at the level of the PAG. Infusion of morphiceptin,
defensive rage. Furthermore, DAME infusion into inhibi-
a m agonist, into the PAG, increased thresholds for PAG-
tory PAG sites inhibited hypothalamically elicited defensive
elicited attack with as low a dose as 0.4 nmol. A smaller
rage. These results suggest that stimulation of inhibitory
increase was observed following infusion of DPDPE, a d
sites in the PAG activated enkephalinergic neurons arising
receptor agonist, but the k agonist U-50488H, had no effect.
from either the central nucleus of the amygdala or from
Furthermore, pretreatment with m and d antagonists, b-
within the PAG itself. Accordingly, infusion of naloxone
Funaltrexamine (b-FNA) and ICI 174864 respectively,
A. Siegel et al. / Neuroscience and Biobehavioral Reviews 23 (1999) 359±389
blocked the suppressive effects of morphiceptin and DPDPE
respectively, indicating the importance of these receptor
subtypes (see Fig. 11).
The third experiment is as follows. It is possible that
opioidergic ®bers constitute interneurons within the PAG
that inhibit defensive rage when activated from an external
source. Alternatively, opioid peptides may be synthesized
in cell bodies located in the amygdala that project to the
PAG, and, when activated, these neurons suppress defen-
sive rage. In order to test this possibility, an experiment
was performed. Initially, stimulation of the central or
lateral nucleus produced a suppression of PAG-elicited
defensive rage which lasted up to 30 min. When non-selec-
tive (naloxone) and selective m (b-FNA) opioid antagonists
were microinjected into PAG defensive rage sites prior to
brain stimulation, the suppressive effects of amygdaloid
stimulation were completely blocked (Fig. 12). However,
a d antagonist (ICI 174864) had no effect. Moreover,
medial amygdaloid facilitation of PAG-elicited defensive
rage was unaffected by infusion of naloxone into the PAG
[192]. These results suggested that the central or lateral
nucleus of the amygdala suppresses defensive rage at the
level of the PAG and that the probable neurotransmitter is
an opioid acting upon m receptors. In order to test whether
the opioid neurons were local interneurons or projection
neurons from another structure, the retrograde tracer
Fluoro-Gold was injected into PAG defensive rage sites.
Cells double-labeled for Fluoro-Gold and met-enkephalin
immunoreactivity were found in the central nucleus and
immediately adjoining regions of the lateral and basal
complex. Notably, cells situated within adjoining parts of
the basal complex of amygdala, which facilitate defensive
rage behavior (see ®g. 21 of Ref. [201], are also double-
labeled for Fluoro-Gold and aspartate or glutamate. Pend-
ing further pharmacological veri®cation, this would
suggest that this region of the basal complex directly facili-
Fig. 12. Naloxone infusion into the PAG completely blocks the suppressive
tates defensive rage behavior at the level of the PAG,
effects of central amygdaloid stimulation upon PAG-elicited defensive rage
utilizing excitatory amino acids as a neurotransmitter.
behavior in a dose-dependent manner. Open circles represent the time
course for amygdaloid-induced suppression of defensive rage behavior as
determined by the percentage change in response latencies from baseline
3.5.1.3. Opioids in the rat. As noted previously, the
values obtained prior to amygdaloid stimulation. These response latencies
were obtained with single stimulation of the PAG following central amyg-
nonspeci®c opioid antagonist naloxone does not affect
daloid stimulation. The latency values returned to baseline levels approxi-
stimulation-induced attacks in the rat [112].
mately 60 min after the last trial of amygdaloid stimulation. Closed circles
indicate the effects of naloxone delivery. Mean data points for each of four
epochs of time (5±30, 30±60, 60±90, and 120±150 min) are represented at
the beginning of each period (with permission).
3.5.2. Cholecystokinin (CCK)
The neuropeptide CCK plays a role in anxiety
responses. Since anxiety resembles defensive rage, in
that both behaviors involve sympathetic arousal in
that infusion of the CCKB antagonist into the PAG
response to a threatening stimulus, one study tested the
facilitated predatory attack.
effect of CCK on defensive rage in the cat [131]. Micro-
injections of the CCKB agonist pentagastrin into the PAG
3.6. Amino acid neurotransmitters
were found to facilitate defensive rage, whereas micro-
injections of the CCKB antagonist, LY288513, but not
3.6.1. Pathways utilizing excitatory amino acids in
the CCKA antagonist, PD140548, suppressed it. The speci-
association with the expression or modulation of aggression
®city of this effect was demonstrated by the observation
Several studies suggest that excitatory amino acids are
A. Siegel et al. / Neuroscience and Biobehavioral Reviews 23 (1999) 359±389
employed to test the hypothesis that the facilitatory effect
of the basal complex of amygdala upon defensive rage is
mediated over a pathway that projects directly to the PAG
and whose functions are mediated by NMDA receptors
[193]. The results showed that the facilitatory effects of
basal amygdaloid stimulation could be reduced by micro-
injections of AP-7 into the PAG. That this NMDA antago-
nist did not block the attack response produced by single
stimulation of the PAG indicates that the observed effects
could not be attributed to anesthetic properties of the drug.
Moreover, this study identi®ed basal amygdaloid neurons
labeled for both glutamate and Fluoro-Gold following
microinjections of the retrograde tracer into attack sites in
the PAG. Collectively, these ®ndings support the view that
basal amygdaloid facilitation of defensive rage is mediated
Fig. 13. Graphs indicate that infusion of kynurenic acid (kyn) into PAG
in part by NMDA receptors over an excitatory amino acid
sites, from which defensive rage behavior can be elicited, blocks the facil-
pathway that projects from the basal amygdala to the PAG.
itatory effects of medial hypothalamic stimulation upon this response
elicited from the PAG. This effect is dose- and time-dependent. Note that
a relatively higher dose of atropine (atr) had little effect upon medial-
3.6.2. Excitatory amino acids and the elicitation of
hypothalamic stimulation-induced aggression. Abbreviation: BL, theoreti-
aggressive responses
cal baseline values indicating the position on the graph where dual stimula-
Local infusion of excitatory amino acids has been utilized
tion of the medial hypothalamus has no effect in altering PAG response
latencies as determined from single stimulation of the PAG. All drug doses
by many investigators to demonstrate that the effects of
are in nanomole values. Vertical bars indicate S.E.M. for this ®gure (with
stimulation upon such events as single unit activity or auto-
nomic processes were the result of activation of cell bodies
rather than ®bers of passage. In recent years, this approach
has been extended to the study of aggressive and related
released at the terminal endings of the ®bers arising from the
emotional processes with mixed success. The most effective
medial hypothalamus and projecting to PAG [129, 182,
region where microinjections of glutamate, aspartate or d,l-
183]. In one experiment, it was demonstrated that the
homocysteic acid can elicit defensive rage behavior is the
nonspeci®c excitatory amino acid antagonist, kynurenic
PAG in the cat [22, 184]. However, defensive rage sites in
acid, microinjected into PAG attack sites, blocked the
the medial hypothalamus are not responsive to microinjec-
medial hypothalamic facilitation of PAG-elicited defensive
tions of excitatory amino acids. In contrast, both electrical
rage, thus supporting the view that excitatory amino acids
and chemical stimulation of the perifornical hypothalamus
act at this synapse (Fig. 13). In additional experiments, infu-
result in the elicitation of predatory attack [22]. In the squir-
sion of the speci®c NMDA antagonist, AP-7, into the PAG,
rel monkey, electrical and chemical stimulation can elicit
blocked medial hypothalamic facilitation, whereas admini-
vocalization at a number of forebrain and brainstem sites,
stration of 6-cyano-7-nitroquinoxaline-2,3-dione (CNQX),
including the lateral hypothalamus, PAG, and lateral
which selectively blocks kainate and quisqualate receptors,
tegmental ®elds of the brainstem [98]. It should be noted
was ineffective. Moreover, infusion of NMDA into the PAG
that the failure to obtain a behavioral response by micro-
facilitated defensive rage elicited from the PAG, suggesting
injection of an excitatory amino acid into a site where elec-
that NMDA receptors mediate the effect. Parallel ®ndings
trical stimulation has elicited the response does not
were observed in a different experiment [182, 183]:
necessarily mean that the response obtained by electrical
hypothalamically elicited defensive rage was blocked
stimulation was the result of stimulation of ®bers of passage.
following microinfusion of AP-7 into the PAG. Finally,
Such a failure may be due to factors such as the diffuse
another study combined retrograde labeling (following
arrangement of cells in the region or the insensitivity of
injections of Fluoro-Gold into the PAG) and immunocyto-
these cells to excitatory amino acids.
chemical methods. Double-labeled cells for both Fluoro-
Gold and aspartate or glutamate were found within the
3.6.3. Excitatory amino acids in the rat See Section 3.8.3.
medial hypothalamus, dorsal and somewhat rostral to the
level of the ventromedial nucleus, thus demonstrating that
3.7. The role of substance P in defensive rage and predatory
glutamatergic neurons project from hypothalamus to PAG.
attack in the cat
These data further serve to support the notion that an exci-
tatory amino acid pathway governs the input to the PAG
Stimulation of the medial amygdala facilitates defensive
from the medial hypothalamus for the expression of defen-
rage elicited from the medial hypothalamus, but suppresses
predatory attack elicited from the lateral hypothalamus
In a separate study, a dual-stimulation paradigm was
[201]. Moreover, the relevant projections from the medial
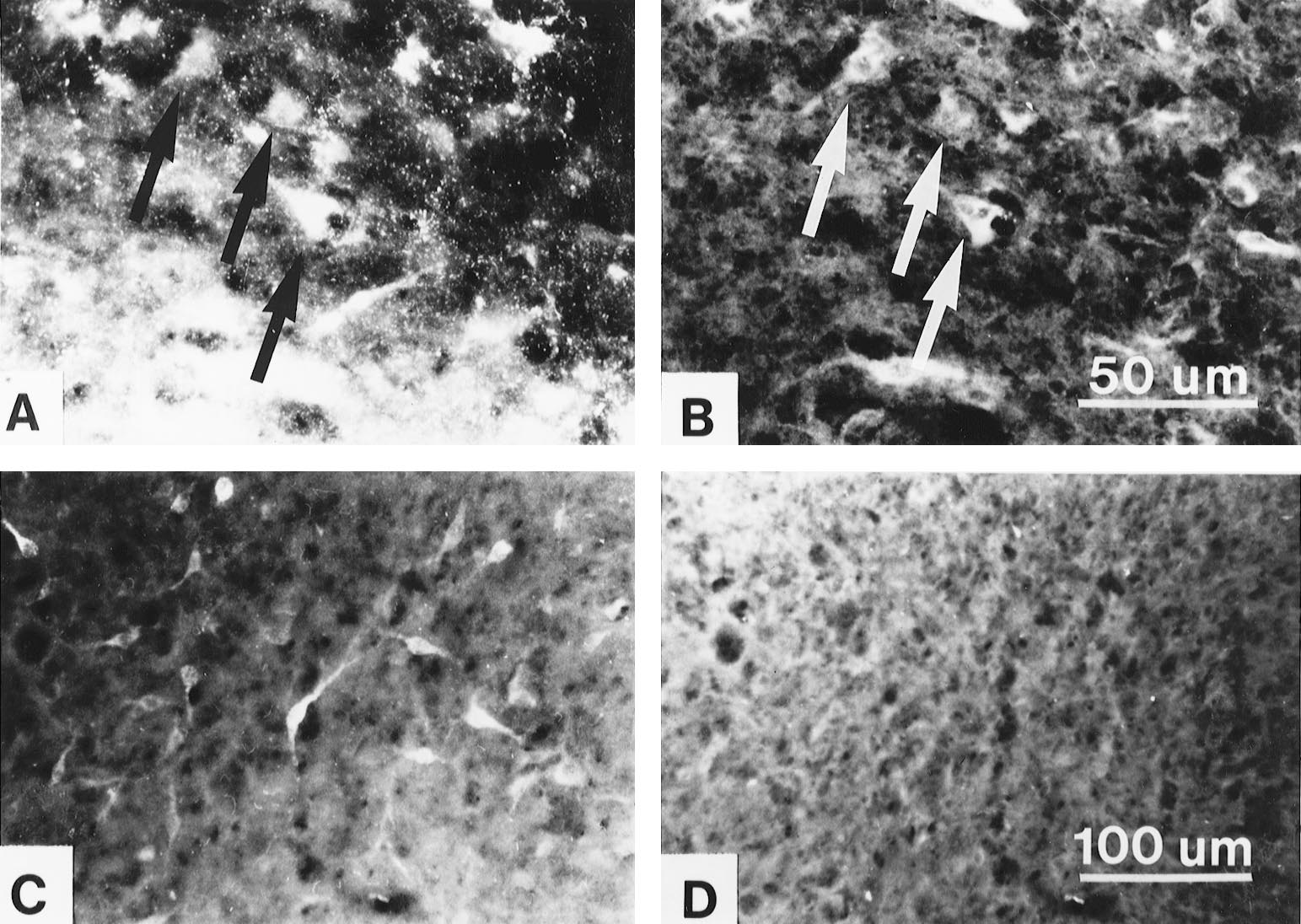
A. Siegel et al. / Neuroscience and Biobehavioral Reviews 23 (1999) 359±389
Fig. 14. (A,C) Microinjections of the retrograde tracer Fluoro-Gold into lateral hypothalamic sites, from which predatory attack behavior could be produced,
resulted in labeled cells within the medial hypothalamus, demonstrating that medial hypothalamic neurons project to the lateral hypothalamus. (B,D)
Immunocytochemical staining for GABA in the same brains showed that GABA-immunopositive neurons are present in the medial hypothalamus. Arrows
in A and B indicate the presence of double-labeled cells, demonstrating that GABAergic neurons project from the medial to lateral hypothalamus. C and D
indicate that some neurons in the medial hypothalamus stain positively only for Fluoro-Gold and not for GABA, suggesting that not all neurons which project
from medial to lateral hypothalamus are GABAergic. (From Ref. [93], with permission.)
amygdala are directed through a monosynaptic pathway (i.e.
hypothalamically elicited predatory attack is also mediated,
the stria terminalis) to the medial hypothalamus [48, 211].
in part, through SP receptors in the medial hypothalamus
This implies that, while the effects of medial amygdaloid
[92]. Speci®cally, microinjections of CP 96,345 into the
stimulation upon defensive rage, which is integrated within
medial hypothalamus blocked medial amygdaloid suppres-
the medial hypothalamus, are direct, the effects upon
sion. However, since the SP pathway mediating suppression
predatory attack, which is integrated within the lateral
of predatory attack terminates in the medial hypothalamus,
hypothalamus, are indirect. The data presented below
such modulation must require at least one additional neuron
provide a likely mechanism that accounts for these effects.
for these effects to take place. The only way to account for
the inhibitory action of the medial amygdala upon predatory
3.7.1. Defensive rage
attack when the initial output neuron from that region is
Medial amygdaloid stimulation facilitates defensive rage
excitatory (i.e. an SP neuron) is to postulate the existence
[48, 70, 211]. In one study, after this ®nding was replicated,
of a second, inhibitory neuron that projects from the medial
the SP-neurokinin 1 antagonist, CP 96,345, was micro-
to the lateral hypothalamus. It was further suggested that
injected into the medial hypothalamus and this was found
such a neuron is likely to utilize GABA as a neurotransmit-
to block the facilitating effects of medial amygdaloid
ter. An experiment undertaken to test this hypothesis is
stimulation [197]. Moreover, following Fluoro-Gold micro-
described below [93].
injections into the medial hypothalamus, neurons labeled for
both SP and Fluoro-Gold were found densely distributed
3.8. Inhibitory amino acids: GABA and glycine
within the medial amygdala but not elsewhere in the
temporal lobe. These observations provided evidence that
3.8.1. Defensive rage
the effects of the medial amygdala upon defensive rage are
GABA serves as an inhibitory neurotransmitter at many
mediated via a monosynaptic pathway that acts upon SP
CNS synapses. In an early study [152], GABA was injected
receptors in the medial hypothalamus.
into medial hypothalamic defensive rage sites. The results,
surprisingly, showed that thresholds were actually lowered
3.7.2. Predatory attack
following drug infusion. In contrast, infusion of glycine into
A sequel to this study, using similar methods, demon-
the same sites resulted in an elevation in thresholds, indicat-
strated that medial amygdaloid suppression of lateral
ing a possible inhibitory role for this putative transmitter.
A. Siegel et al. / Neuroscience and Biobehavioral Reviews 23 (1999) 359±389
In a later study [196] cannula-electrodes were implanted
GABAA receptors in the lateral hypothalamus, where inte-
into defensive rage sites within the dorsal PAG. Microinjec-
gration of predatory attack occurs.
tions of the GABA agonist muscimol into defensive rage
Most recently, a study [55] using a retrograde tracer and
sites within the dorsal PAG produced elevations in threshold
immunocytochemical labeling for GABA showed that the
at as low a dose as 12 pmol. Muscimol had no effect upon
inhibitory projection from medial to lateral hypothalamus is
predatory attack responses elicited from ventral PAG. In
matched by a reciprocal GABAergic projection from lateral
addition, pretreatment with bicuculline, a GABAA
to medial hypothalamus. This study also found that bicucul-
antagonist, completely blocked the suppressive effects of
line antagonism in the medial hypothalamus did not facili-
muscimol [196]. Thus, it appears that GABA selectively
tate defensive rage elicited by medial hypothalamic
modulates defensive rage by serving as an inhibitory trans-
stimulation alone; however, bicuculline blocked the
mitter within the PAG. Nevertheless, a number of questions
suppressive effects of lateral hypothalamic stimulation
remain unanswered with respect to the overall role of
upon defensive rage elicited by stimulation of the medial
GABA in the PAG. For example, we do not know the speci-
hypothalamus. The overall ®ndings of these studies are
®c receptor subtype(s) involved; nor do we know the origins
summarized in Fig. 10.
of these GABA neurons. Are they local interneurons within
The discovery of these reciprocal inhibitory pathways
a single functional column of the PAG [23]? Or do they
gives a neuroanatomical basis for the longstanding observa-
originate from adjacent columns, or from distant regions
tion that manipulations that inhibit defensive rage will also
of the forebrain and brainstem, and speci®cally project to
facilitate predatory attack, and vice versa. For example,
sites within the PAG that mediate defensive rage behavior?
Adamec [2±6] and Adamec and Stark-Adamec [7] observed
Answers to these questions would help us understand how
that limbic kindling involved, in part, neurons of origin of
GABA affects defensive rage and related forms of
the stria terminalis, a tract which carries ®bers from the
emotional behavior.
amygdala to medial hypothalamus. Kindling of these sites
caused changes in the behavioral repertoire of the catsÐ
3.8.2. Predatory attack and defensive rage: a reciprocal
speci®cally, they became more defensive and less prone to
inhibitory pathway between the medial hypothalamus and
display predatory responses. If this kindling directly facili-
lateral hypothalamus of the cat
tated neuronal activity within the medial hypothalamus as a
As indicated above, it was hypothesized that medial
result of activation of the stria terminalis, then such excita-
amygdaloid suppression of lateral-hypothalamically elicited
tion would have enhanced functions associated with the
predatory attack was mediated through a GABAergic
medial hypothalamus, such as defensive behavior. Congru-
neuron that projects from the medial to lateral hypothala-
ently, the observed reduction in predatory responses may
mus. To test this hypothesis [93], suppression of lateral-
have been due to inhibition of the lateral hypothalamus by
hypothalamically elicited predatory attack was induced
activation of the short GABAergic projection from the
either by electrical stimulation of the medial amygdala or
by microinjection of SP into the medial hypothalamus.
Suppression of predatory attack induced by either of these
3.8.3. GABA in the rat
procedures could be blocked following microinjections of
Adams et al. [9] and Roeling et al. [174] gave evidence
the selective GABAA antagonist, bicuculline, into the lateral
for involvement of GABA receptors in hypothalamic
hypothalamus. Moreover, this study also demonstrated the
aggression in rats. Local infusion of the GABAA antagonist
presence of GABA receptors within the lateral hypothala-
bicuculline induced attacks, [174], and the GABA antago-
mus as well as neurons in the medial hypothalamus that
nist picrotoxin also induced aggression [9]. Most recently
were labeled for both Fluoro-Gold and GABA immunoreac-
Haller et al. [91] elicited aggression in the hypothalamus
tivity following microinjections of the retrograde tracer into
with a mixture of glutamate agonist and bicuculline applied
the lateral hypothalamus (Fig. 14).
by microdialysis, but this mixture seemed to work only in
The results of this study, coupled with the data described
above, account for the differential effects of medial amyg-
daloid stimulation on defensive rage and predatory attack.
3.9. Effects of administration of tranquilizers,
In summary, the medial amygdaloid facilitation of defen-
antidepressants and psychotropic drugs upon stimulation-
sive rage is mediated through a monosynaptic, SP pathway
induced aggressive reactions
directly upon the medial hypothalamus, where integration of
this form of aggression occurs. The suppressive effects of
A number of investigators have attempted to determine
the medial amygdala upon predatory attack are mediated
how tranquilizers, antidepressants and psychotropic drugs
over a disynaptic pathway. The ®rst limb of this pathway
affect the aggression elicited by brain stimulation, using
constitutes the (excitatory) SP projection from the medial
various strategies and objectives. In one approach, aggres-
amygdala to medial hypothalamus, and the second limb
sive behavior is measured as part of a battery of behavioral
consists of a GABAergic projection from the medial to
and physiological tests in order to assess the ef®cacy of a
lateral hypothalamus whose functions are mediated by
number of different compounds. In this situation, the
A. Siegel et al. / Neuroscience and Biobehavioral Reviews 23 (1999) 359±389
principal concern is to characterize the properties of the
generally increased, while predatory behavior was
drugs for purposes of clinical applications. In a second
suppressed. Moreover, the effects of FG-7142 upon defen-
approach, drugs are employed as tools in order to study
sive behavior were reversed following administration of the
how speci®c transmitters affect aggression. In general,
benzodiazepine antagonist, ¯umazenil. An interesting
when positive results are obtained from drug tests, the
feature of these studies was that they were able to show
drugs are likely to appear to suppress aggression. The
that FG-7142 enhanced the evoked potential in the medial
primary dif®culty in interpreting such results is that it may
hypothalamus resulting from basal amygdaloid stimula-
not be possible to determine whether such effects are beha-
tion. Assuming that this evoked potential was mediated
viorally speci®c to aggression or extend to a wider range of
over the stria terminalis, it is reasonable to assume that
behavioral (motor) responses; few studies have addressed
potentiation of this response by FG-7142 resulted from its
actions upon medial and adjoining basal amygdaloid
Early studies indicated that thresholds for defensive rage
neurons which comprise the origin of the stria terminalis.
elicited from the medial hypothalamus of the cat are
Furthermore, the differential effects of FG-7142 upon
elevated following administration of antidepressants such
defensive and predatory behavior can be understood in
as imipramine, desimpramine and amitryptyline [30, 79].
terms of the circuitry from the medial amygdala to the
It should be noted that imipramine and desimpramine also
medial and lateral hypothalamus that was elaborated
attenuate predatory attack responses in the cat [69]. In parti-
above. Said otherwise, if FG-7142 produces its effects
cular, one study [68] observed that imipramine had little or
by driving neurons that comprise the origins of the stria
no effect upon defensive rage in the cat and another [164]
terminalis, then activation of these neurons would excite
found differential dose effects: a low dose (2.5 mg/kg)
medial hypothalamic neurons, which would increase the
facilitated defensive rage and a higher dose (8±10 mg/kg)
likelihood of occurrence of defensive rage. At the same
suppressed this response. However, the clinically effective
time, activation of medial hypothalamic neurons would,
antidepressant ¯uvoxamine has signi®cant effects on
by virtue of a GABAergic interneuron, suppress lateral
hypothalamic attack in the rat [112, 118, 158].
hypothalamic neurons that mediate predatory attack.
Considerable attention has been given to the benzodiaze-
Additional studies are required to identify the neuro-
pine drugs, which act upon the GABA receptor complex.
physiological effects of FG-7142 treatment upon neurons
Investigators have utilized chlordiazepoxide in several
in the medial and basal amygdala and medial and lateral
species. In the rat [162], cat [28, 79, 137] and monkey
[67], peripheral administration resulted in a suppression of
Ethanol, which may act upon GABA receptors, has been
defensive rage and ®ghting responses. However, in the rat, a
reported to affect defensive rage oppositely to the benzodia-
study reported that chlordiazepoxide facilitated the occur-
zepines. Speci®cally, ethanol was shown to facilitate defen-
rence of quiet biting attack'' in the rat [162], whereas
sive rage and suppress predatory attack in cats (Ref. [182]
another study reported that it affects lateral threat more
reviewed in Ref. [207]).
than biting or ®ghting [118]. It appears that, in rat, benzo-
diazepines only have effects at the highest doses, where the
muscle relaxant properties of these drugs are very
4. Summary and conclusions
pronounced (Table 2 and Ref. [118]).
Shaikh et al. [189] observed that carbamazepine selec-
The major achievements of the research carried out to
tively suppressed PAG-elicited defensive rage in cats
date have been to characterize the functional anatomy and
while having no effect upon PAG-elicited predatory attack.
pharmacology of the synaptic regions critical for the expres-
By comparison, in rats, carbamazepine has no effect on
sion and modulation of aggressive responses. Of particular
hypothalamic attack [118]. Similar ®ndings with respect
signi®cance have been the studies in which selective
to medial-hypothalamically elicited defensive rage were
agonists and antagonists were administered to speci®c
observed with diazepam, oxazepam [137], etizolam [78]
receptor populations involved in the expression and modu-
and Y-7131, an experimental drug [215]. Suppressive
lation of aggression.
effects upon defensive rage were also noted for such anti-
Although the anatomical relationships governing aggres-
anxiety drugs as chlorpromazine and pentobarbital [30,
sion in the rat have yet to be elucidated, existing data
134]. Thus, the overall effects of benzodiazepines upon
suggest the presence of similarities as well as differences
cat defensive rage are inhibitory, paralleling the ®ndings
between the rat and cat. One similarity is that for both
obtained with GABA agonists.
species, the hypothalamus seems to be critical for the
Recently, a series of papers offered an alternative
expression of aggressive responses; and the attack mechan-
approach to the study of benzodiazepines and aggression
ism seems to be powerfully modulated by different nuclei of
[2, 5, 6]. These studies showed that when the anxiogenic
the amygdala. Also, emerging data suggest that, for both
inverse benzodiazepine agonist FG-7142 (N-methyl-b-
species, serotonergic mechanisms play an inhibitory role
carboline 3 carboxamide), which is a b-carboline, is
in the regulation of aggression. The two species differ
systemically administered to cats, defensive behavior was
with respect to the organization of the response systems.
A. Siegel et al. / Neuroscience and Biobehavioral Reviews 23 (1999) 359±389
Speci®cally, in the cat, two distinct descending pathways
methods as more precise anterograde and retrograde tract
from the hypothalamus to the PAG and other parts of the
tracing procedures with immunocytochemistry and neuro-
brainstem mediate the expression of defensive rage and
pharmacology will ultimately provide us with a better
predatory attack. However, in the rat, such a medial-to-
means of verifying the nature of the transmitters as well
lateral distinction for separate response systems is not
as the origin and distribution of the pathways under inves-
apparent. In addition, while the PAG is critical for the
tigation. Methods for measurement of transmitter release,
execution of aggression and defense in the cat, its role in
such as in vivo microdialysis, should aid in clarifying the
rodent aggression is unclear.
nature of the transmitters released at key synapses during
That this research is relevant to human disorders is illu-
the expression of an attack response. Likewise, in situ
strated by the following examples. In humans, low serotonin
hybridization and other histochemical methods will further
has been implicated in violent murder and suicide [21, 44,
help to identify the properties of neurons and neurotrans-
59±62, 181]. Moreover, recently a genetic trait in humans,
mitter receptors under investigation.
associated with abnormal serotonin metabolism and person-
ality disturbances including aggressive dyscontrol, has been
described [47], and mimicked in mice in which the gene for
monoamine oxidase A was knocked out [54]. Moreover,
reuptake inhibitors of serotonin like clomipramine, ¯uoxe-
This research was supported, in part, by NIH grant NS
tine and ¯uvoxamine are therapeutically effective in obses-
07941-27, the Foundation of the University of Medicine and
sive±compulsive disorders that escape control of the central
Dentistry of New Jersey, and the Alcoholic Beverage Medi-
mechanisms involved in the assessment of response appro-
cal Research Foundation (to A Siegel). Another part of this
priateness [168]. In addition, propranolol is effective in the
study was supported by the Foundation for Biological
control of pathological aggression [199], and oxazepam is
Research (BION), which is subsidized by the Netherlands
more effective than chlordiazepoxide in reducing feelings of
Organisation for Scienti®c Research (NWO) (Grants no.
hostility in humans [127]. These pharmacological and func-
430.901P and 430.902P) to TAP Roeling and MR Kruk.
tional parallels suggest that studying the ethology, physiol-
The authors wish to thank the Harry Frank Guggenheim
ogy and pharmacology of hypothalamic responses may
Foundation which provided separate grant support to A
facilitate the understanding of the pathophysiology of
Siegel and MR Kruk. The authors are indebted to Dr. KA
human behavioral disorders.
Miczek, Dr. B Olivier and Dr. AM Van der Poel for their
From the data presented here, it would appear that the
support and review of the manuscript.
central mechanisms involved in aggression are species-
speci®c to a great extent. Such species differences in aggres-
sion between a highly specialized carnivorous species (i.e.
cat) and an opportunistic omnivorous species (i.e. rat) have
been postulated on functional grounds in classical ethologi-
[1] Aalders TTA, Meek J. The hypothalamic aggression region of the
rat: observations on the synaptic organization. Brain Res Bull
cal studies [128].
In retrospect, although much data has been accumulated
[2] Adamec R. Transmitter systems involved in neural plasticity under-
concerning the neurobiology of aggression over the past
lying increased anxiety and defenseÐimplications for understand-
25 years, an overall understanding of the nature of the
ing anxiety following traumatic stress. Neurosci Biobehav Rev
neurotransmitters regulating aggression has still not been
[3] Adamec RE. Role of the amygdala and medial hypothalamus in
achieved. Our capacity to identify precisely speci®c trans-
spontaneous feline aggression and defense. Aggress Behav
mitters at key synapses along the pathways associated with
the expression or modulation of the attack responses has
[4] Adamec RE. Partial kindling of the ventral hippocampus: identi®ca-
been limited by available methodologies as well as by de®-
tion of changes in limbic physiology which accompany changes in
cits in our knowledge of the functional neuroanatomy of
feline aggression and defense. Physiol Behav 1991;49:443±453.
[5] Adamec RE. Lasting effects of FG-7142 on anxiety, aggression and
aggression. Examples of some of the technical limitations
limbic physiology in the cat. J Psychopharmacol 1993;7:232±248.
include: (1) the unavailability of highly selective receptor
[6] Adamec RE. Partial limbic kindlingÐbrain, behavior, and the
subtype agonists and antagonists that are required for appro-
benzodiazepine receptor. Physiol Behav 1993;54:531±545.
priate testing of given transmitter systems; (2) lack of appro-
[7] Adamec RE, Stark-Adamec C. Behavioral inhibition and anxiety:
priate neuroanatomical tools to identify precisely the
dispositional, developmental, and neural aspects of the anxious
personality of the domestic cat. In: Resnick JS, editor. Perspectives
pathways as well as synaptic relationships governing the
on behavioral inhibition. Chicago, IL: University of Chicago Press,
functional pathways in question; (3) our general lack of
precision in measuring transmitter release at given synapses
[8] Adams DB. Defense and territorial behaviour dissociated by
in association with the expression of a speci®c form of
hypothalamic lesions in the rat. Nature 1971;232:573±574.
attack behavior.
[9] Adams DB, Boudreau W, Cowan CW, Kokonowski C, Oberteuffer
K, Yohay K. Offense produced by chemical stimulation of the ante-
On the positive side, some of these limitations are in the
rior hypothalamus of the rat. Physiol Behav 1993;53:1127±1132.
process of being overcome. Our ability to combine such
[10] Albert DJ, Brayley KN. Mouse killing and hyperreactivity following
A. Siegel et al. / Neuroscience and Biobehavioral Reviews 23 (1999) 359±389
lesions of the medial hypothalamus, the lateral septum, the bed
[31] Beleslin DB, Samardzic R. The pharmacology of aggressive beha-
nucleus of the stria terminalis, or the region ventral to the anterior
vioural phenomena elicited by muscarine injected into the cerebral
septum. Physiol Behav 1979;23:439±443.
ventricles of conscious cats. Psychopharmacology (Berl)
[11] Albert DJ, Brayley KN, Milner JA. Medial hypothalamic electrical
stimulation is ineffective in suppressing septal lesion induced hyper-
[32] Berger BD, Schuster R. Pharmacological aspects of social coopera-
reactivity. Physiol Behav 1978;21:135±139.
tion. In: Olivier B, Mos J, Brain PF, editors. Ethopharmacology of
[12] Albert DJ, Nanji N, Brayley KN, Madryga FJ. Hyperreactivity as
agonistic behaviour in humans and animals. Dordrecht: Martinus
well as mouse killing is induced by electrical stimulation of the
lateral hypothalamus in the rat. Behav Neural Biol 1979;27:59±71.
[33] Bermond B, Mos J, Meelis W, Van der Poel AM, Kruk MR. Aggres-
[13] Albert DJ, Walsh ML. Neural systems and the inhibitory modulation
sion induced by stimulation of the hypothalamus: effects of andro-
of agonistic behavior: a comparison of mammalian species. Neurosci
gens. Pharmacol Biochem Behav 1982;16:41±45.
Biobehav Rev 1984;8:5±24.
[34] Berntson GG. Attack, grooming, and threat elicited by stimulation of
[14] Albert DJ, Walsh ML, White R, Longley W. A comparison of prey
the pontine tegmentum in cats. Physiol Behav 1973;11:81±87.
eating by spontaneous mouse killing rats and rats with lateral septal,
[35] Berntson GG, Beattie MS, Walker JM. Effects of nicotinic and
medial accumbens, or medial hypothalamic lesions. Physiol Behav
muscarinic compounds on biting attack in the cat. Pharmacol
Biochem Behav 1976;5:235±239.
[15] Albert DJ, White R, Walsh ML. Rearing rats with mice prevents
[36] Berntson GG, Hughes HC, Beattie MS. A comparison of hypotha-
induction of mouse killing by lesions of the septum but not lesions of
lamically induced biting attack with natural predatory behavior in
the medial hypothalamus or medial accumbens. Physiol Behav
the cat. The Journal of Comparative and Physiological Psychology
[16] Albert DJ, Wong RCK. Hyperreactivity, muricide, and intraspeci®c
[37] Berntson GG, Leibowitz SF. Biting attack in cats: evidence for
aggression in the rat produced by infusion of local anesthetic into the
central muscarinic mediation. Brain Res 1973;51:366±370.
lateral septum or surrounding areas. J Comp Physiol Psychol
[38] Blanchard DC, Blanchard RJ. Aggressive behavior in the rat. Beha-
vioral Biology 1977;21:197±224.
[17] Allikmets LH. Cholinergic mechanisms in aggressive behaviour.
[39] Blanchard DC, Blanchard RJ, Fukunaga K, Kelley MJ. Conspeci®c
Medical Biol 1974;52:19±30.
aggression in the laboratory rat. J Comp Physiol Psychol
[18] Allikmets LH, Vahing VA, Lapin IP. Dissimilar in¯uences of
imipramine, benactyzine and promazine on effects of micro-injec-
[40] Blanchard DC, Blanchard RJ, Takahashi T, Kelley MJ. Attack and
tions of noradrenaline, acetylcholine and serotonin into the amyg-
defensive behaviour in the albino rat. Anim Behav 1977;25:622±
dala in the cat. Psychopharmacologia 1969;15:392±403.
[19] Andy OJ, Velamati S. Limbic system seizures and aggressive beha-
[41] Blanchard RJ, Blanchard DC. The organization and modeling of
vior (superkindling effects). Pav J Biol Sci 1978;13:251±264.
animal aggression. In: Brain PF, Benton D, editors. The biology of
[20] Atweh SF, Kuhar MJ. Autoradiographic localization of opiate recep-
aggression. Alphen aan den Rijn: Sijthoff and Noordhoff, 1981:529±
tors in rat brain. III. The telencephalon. Brain Res 1977;134:393±
[42] Block CH, Siegel A, Edinger H. Effects of amygdaloid stimula-
[21] AÊsberg M, TraÈskman L, ThoreÂn P. 5-HIAA in the cerebrospinal
tion upon trigeminal sensory ®elds established during hypothala-
¯uid: a biochemical suicide predictor. Arch Gen Psychiatry
mically-elicited quiet biting attack in the cat. Brain Res
[22] Bandler R. Identi®cation of hypothalamic and midbrain periaque-
[43] Brady JV, Nauta WJH. Subcortical mechanisms in emotional beha-
ductal grey neurones mediating aggressive and defensive behaviour
vior: the duration of affective changes following septal and habenu-
by intracerebral microinjections of excitatory amino acids. In: Band-
lar lesions in the albino rat. J Comp Physiol Psychol 1954;48:412±
ler R, editor. Modulation of sensorimotor activity during alterations
in behavioral states. New York: Alan R Liss, 1984:369±91.
[44] Brown GL, Ebert MH, Goyer PF, Jimerson DC, Klein WJ, Bunney
[23] Bandler R, Shipley MT. Columnar organization in the midbrain
WE, Goodwin FK. Aggression, suicide, and serotonin: relation-
periaqueductal gray: modules for emotional expression. Trends
ships to CSF amine metabolites. Am J Psychiatry 1982;139:741±
[24] Bandler R, Tork I. Midbrain periaqueductal grey region in the cat
[45] Brudzynski SM. Carbachol-induced agonistic behavior in cats:
has afferent and efferent connections with solitary tract nuclei.
aggressive or defensive response? Acta Neurobiol Exp
Neurosci Lett 1987;74:1±6.
[25] Bandler RJ, Chi CC, Flynn JP. Biting attack elicited by stimulation
[46] Brudzynski SM. Growling component of vocalization as a quantita-
of the ventral midbrain tegmentum of cats. Science 1972;177:364±
tive index of carbachol-induced emotional±defensive response in
cats. Acta Neurobiol Exp 1981;41:33±51.
[26] Barrett J, Edinger H, Siegel A. Intrahypothalamic injections of nore-
[47] Brunner HG, Nelen MR, Van Zandvoort P, Abeling NGGM, Van
pinephrine facilitate feline affective aggression via alpha-2 adreno-
Gennip AH, Wolters EC, Kuiper MA, Ropers HH, Van Oost BA. X-
ceptors. Brain Res 1990;525:285±293.
linked borderline mental retardation with prominent behavioural
[27] Barrett JA, Shaikh MB, Edinger H, Siegel A. The effects of intra-
disturbance: phenotype, genetic localization, and evidence for
hypothalamic injections of norepinephrine upon affective defense
disturbed monoamine metabolism. Am J Hum Genet
behavior in the cat. Brain Res 1987;426:381±384.
[28] Baxter BL. The effect of chlordiazepoxide on the hissing
[48] Brutus M, Shaikh MB, Siegel A, Edinger H. Effects of experimental
response elicited via hypothalamic stimulation. Life Sci
temporal lobe seizures upon hypothalamically elicited aggressive
behavior in the cat. Brain Res 1986;366:53±63.
[29] Baxter BL. Elicitation of emotional behavior by electrical or chemi-
[49] Brutus M, Shaikh MB, Siegel A, Siegel HE. An analysis of the
cal stimulation applied at the same loci in cat mesencephalon. Exp
mechanisms underlying septal area control of hypothalamically
elicited aggression in the cat. Brain Res 1984;310:235±248.
[30] Baxter BL. The effect of selected drugs on the emotional'' beha-
[50] Brutus M, Siegel A. Effects of the opiate antagonist naloxone upon
vior elicited via hypothalamic stimulation. Int J Neuropharmacol
hypothalamically elicited affective defense behavior in the cat.
Behav Brain Res 1989;33:23±32.
A. Siegel et al. / Neuroscience and Biobehavioral Reviews 23 (1999) 359±389
[51] Brutus M, Zuabi S, Siegel A. Effects of d-ala2-met5-enkephalina-
[73] Flynn JP, MacDonnell MF. Control of sensory ®elds by stimulation
mide microinjections placed into the bed nucleus of the stria termi-
of hypothalamus. Science 1966;152:1406±1408.
nalis upon affective defense behavior in the cat. Brain Res
[74] Flynn JP, Vanegas H, Foote WE, Edwards S. Neural mechanisms
involved in a cat's attack on a rat. In: Whalen R, editor. The neural
[52] Brutus M, Zuabi S, Siegel A. Microinjection of d-ala2-met5-enke-
control of behavior. New York: Academic Press, 1970:135±73.
phalinamide placed into the nucleus accumbens suppresses feline
[75] Fuchs SAG, Dalsass M, Siegel HE, Siegel A. The neural pathways
affective defense behavior. Exp Neurol 1989;104:55±61.
mediating quiet-biting attack behavior from the hypothalamus in the
[53] Carlsson A, Corrodi H, Fuxe K, Hokfelt T. Effect of antidepressant
cat: a functional autoradiographic study. Aggress Behav 1981;7:51±
drugs on the depletion of intraneuronal brain 5-hydroxytrystamine
stores caused by 4-methyl-alpha-ethyl-meta-tyramine. Eur J Phar-
[76] Fuchs SAG, Edinger HM, Siegel A. The organization of the
hypothalamic pathways mediating affective defense behavior in
[54] Cases O, Seif I, Grimsby J, Gaspar P, Chen K, Pournin S, MuÈller U,
the cat. Brain Res 1985;330:77±92.
Aguet M, Babinet C, Shih JC, De Maeyer E. Aggressive behavior
[77] Fuchs SAG, Edinger HM, Siegel A. The role of the anterior hypotha-
and altered amounts of brain serotonin and norepinephrine in mice
lamus in affective defense behavior elicited from the ventromedial
lacking MAOA. Science 1995;268:1763±1766.
hypothalamus of the cat. Brain Res 1985;330:93±108.
[55] Cheu JW, Siegel A. GABA receptor mediated suppression of defen-
[78] Fukuda T, Tsumagari T. Effects of psychotropic drugs on the rage
sive rage behavior elicited from the medial hypothalamus of the cat:
responses induced by electrical stimulation of the medial hypotha-
role of the lateral hypothalamus. Brain Res 1998;783:293±304.
lamus in cats. Jpn J Pharmacol 1983;33:885±890.
[56] Chi CC, Flynn JP. Neural pathways associated with hypothalami-
[79] Funderburk WH, Foxwell MH, Hakala MW. Effects of psychother-
cally elicited attack behavior in cats. Science 1971;171:703±706.
apeutic drugs on hypothalamic-induced hissing in cats. Neurophar-
[57] Chi CC, Flynn JP. Neuroanatomic projections related to biting attack
elicited from hypothalamus in cats. Brain Res 1971;35:49±66.
[80] Gall C, Moore RY. Distribution of enkephalin, substance P, tyrosine
[58] Clark FM, Proud®t HK. The projection of locus coeruleus neurons to
hydroxylase, and 5-hydroxytryptamine immunoreactivity in the
the spinal cord in the rat determined by anterograde tracing
septal region of the rat. J Comp Neurol 1984;225:212±227.
combined with immunocytochemistry. Brain Res 1991;538:231±
[81] Geeraedts LMG, Nieuwenhuys R, Veening JG. Medial forebrain
bundle of the rat: III. Cytoarchitecture of the rostral (telencephalic)
[59] Coccaro EF. Central serotonin and impulsive aggression. Br J
part of the medial forebrain bundle bed nucleus. J Comp Neurol
Psychiatry 1989;155(Suppl 8):52±62.
[60] Coccaro EF. The biology of aggression. Sci Am 1995;266:38±47.
[82] Geeraedts LMG, Nieuwenhuys R, Veening JG. Medial forebrain
[61] Coccaro EF, Gabriel S, Siever LJ. Buspirone challenge: preliminary
bundle of the rat: IV. Cytoarchitecture of the caudal (lateral hypotha-
evidence for a role for central 5HT-1a receptor function in impulsive
lamic) part of the medial forebrain bundle bed nucleus. J Comp
aggressive behavior in humans. Psychopharmacol Bull 1990;26:405.
[62] Coccaro EF, Siever LJ, Klar HM, Maurer G, Cochrane K, Cooper
[83] George R, Haslett WL, Jenden DJ. The central action of a metabolite
TB, Mohs RC, Davis KL. Serotonergic studies in patients with
of tremorine. Life Sci 1962;8:361±363.
affective and personality disorders. Arch Gen Psychiatry
[84] Geyer M, Segal D. Shock-induced aggression: opposite effects of
intraventricularly infused dopamine and norepinephrine. Behavioral
[63] Crescimanno G, Piazza P, Benigno A, Amato G. Effects of substan-
tia nigra stimulation on hypothalamic rage reaction in cats. Physiol
[85] Glennon RA, Pierson ME, McKenney JD. Stimulus generalization
of 1-(3-tri¯uoromethylphenyl) piperazine (TFMPP) to propanolol,
[64] Dalsass M, Siegel A. Opioid peptide regulation of neurons in the bed
nucleus of the stria terminalis: a microiontophoretic study. Brain Res
[86] Golebiewski H, Romaniuk A. The participation of serotoninergic
[65] de Bruin JPC. Orbital prefrontal cortex, dopamine, and social
system in the regulation of emotional±defensive behavior evoked
agonistic behavior of male Long Evans rats. Aggress Behav
by intrahypothalamic carbachol injections in the cat. Acta Neurobiol
Exp 1985;45:25±36.
[66] De Kloet ER, Korte SM, Rots NY, Kruk MR. Stress hormones,
[87] Goodman RR, Snyder SH, Kuhar MJ, Young WS. Differentiation
genotype and brain organization: implications for aggression. Ann
of delta and mu opiate receptor localizations by light
N Y Acad Sci 1996;794:179±191.
microscopic autoradiography. Proc Natl Acad Sci USA
[67] Delgado JMR. Antiaggressive effects of chlordiazepoxide. In: Garat-
tini S, Mussini E, Randall LO, editors. The benzodiazepines. New
[88] Green AR, Grahame-Smith DG. Propanolol inhibits the behavioural
York: Raven Press, 1973:419±32.
responses of rats to increased 5-hydroxytryptamine in the central
[68] Dubinsky B, Goldberg ME. The effect of imipramine and selected
nervous system. Nature 1976;262:594±596.
drugs on attack elicited by hypothalamic stimulation in the cat.
[89] Gros C, Pradelles P, Humbert J, Dray F, Le Gal La Salle G, Ben-Ari
Y. Regional distribution of met-enkephalin within the amygdaloid
[69] Dubinsky B, Karpowicz JK, Goldberg ME. Effects of tricyclic anti-
complex and bed nucleus of the stria terminalis. Neurosci Lett
depressants on attack elicited by hypothalamic stimulation: relation
to brain biogenic amines. J Pharmacol Exp Ther 1973;187:550±557.
[90] Haccou P, Kruk MR, Meelis E, Van Bavel ET, Wouterse KM,
[70] Egger MD, Flynn JP. Effects of electrical stimulation of the amyg-
Meelis W. Markov models for social interactions: analysis of elec-
dala on hypothalamically elicited attack behavior in cats. J Neuro-
trical stimulation in the hypothalamic aggression area of rats. Anim
[71] Ferguson J, Henriksen S, Cohen H, Mitchell G, Barchas J, Dement
[91] Haller J, Kruk MR, Makara GB. Three levels of catecholaminergic
W. Hypersexuality'' and behavioral changes in cats caused by
control over aggression: the hormones, the sympathetic and the
administration of p-chlorophenylalanine. Science 1970;168:499±
central noradrenergic systems. Neurosci Biobehav Rev (in press).
[92] Han Y, Shaikh MB, Siegel A. Medial amygdaloid suppression of
[72] Fernandez De Molina A, Hunsperger RW. Organization of the
predatory attack behavior in the cat: I. Role of a substance P pathway
subcortical system governing defence and ¯ight reactions in the
from the medial amygdala to the medial hypothalamus. Brain Res
cat. J Physiol 1962;160:200±213.
A. Siegel et al. / Neuroscience and Biobehavioral Reviews 23 (1999) 359±389
[93] Han Y, Shaikh MB, Siegel A. Medial amygdaloid suppression of
stimulation in the hypothalamus of male CPBWEzob and CPBWI
predatory attack behavior in the cat: II. Role of a GABAergic path-
rats. Aggress Behav 1990;16:177±190.
way from the medial to the lateral hypothalamus. Brain Res
[116] Kruk MR, Van der Poel AM. Is there evidence for a neural correlate
of an aggressive behavioural system in the hypothalamus of the rat?
[94] Hernandez-Peon R, Chavez-Ibarra G, Morgane PJ, Timo-Iaria C.
Prog Brain Res 1980;53:385±390.
Limbic cholinergic pathways involved in sleep and emotional beha-
[117] Kruk MR, Van der Poel AM, De Vos-Frerichs TP. The induction of
vior. Exp Neurol 1963;8:93±111.
aggressive behaviour by electrical stimulation in the hypothalamus
[95] Herndon JG, Adrian AP, McCoy M. Orthogonal relationship
of male rats. Behaviour 1979;70:292±322.
between electrically elicited social aggression and selfstimulation
[118] Kruk MR, Van der Poel AM, Lammers JHCM, Hagg T, de Hey
from the same brain sites. Brain Res 1979;171:374±380.
AMDM, Oostwegel S. Ethopharmacology of hypothalamic
[96] Hess WR, Brugger M. Das subkortikale Zentrum der affektiven
aggression in the rat. In: Olivier B, Mos J, Brain PF, editors. Etho-
Abwehrreaktion. Helv Physiol Pharmacol Acta 1943;1:33±52.
pharmacology of agonistic behaviour in animals and humans.
[97] Holstege G, Meiners L, Tan K. Projections of the bed nucleus of the
Dordrecht: Martinus Nijhoff, 1987:35±45.
stria terminalis to the mesencephalon, pons, and medulla oblongata
[119] Kruk MR, Van der Poel AM, Meelis W, Hermans J, Mostert PG,
in the cat. Exp Brain Res 1985;58:379±391.
Mos J, Lohman AHM. Discriminant analysis of the localization of
[98] Jurgens U, Richter K. Glutamate-induced vocalization in the squirrel
aggression-inducing electrode placements in the hypothalamus of
monkey. Brain Res 1986;373:349±358.
male rats. Brain Res 1983;260:61±79.
[99] Karmos-Varszegi M, Karmos G. A comparative study of autonomic,
[120] Lammers JHCM, Kruk MR, Meelis W, Van der Poel AM. Hypotha-
somatic and bioelectric correlates of emotional reactions elicited by
lamic substrates for brain stimulation-induced attack, teeth-chatter-
cholinergic stimulation of the hypothalamus and the ventral tegmen-
ing and social grooming in the rat. Brain Res 1988;449:311±327.
tum. Activ Nerv Sup 1977;19:2.
[121] Lammers JHCM, Kruk MR, Meelis W, Van der Poel AM. Hypotha-
[100] Katz RJ, Thomas E. Effects of scopolamine and alpha-methylpara-
lamic substrates for brain stimulation-induced patterns of locomo-
tyrosine upon predatory attack in cats. Psychopharmacologia
tion and escape jumps in the rat. Brain Res 1988;449:294±310.
[122] Lammers JHCM, Meelis W, Kruk MR, Van der Poel AM. Hypotha-
[101] Kemble ED. Some further ethoexperimental studies of the anti-
lamic substrates for brain stimulation-induced grooming, digging
aggressive drug ¯uprazine hydrochloride. In: Blanchard RJ, Brain
and circling in the rat. Brain Res 1987;418:1±19.
PF, Blanchard DC, Parmigiani S, editors. Ethoexperimental
[123] Lammers JHCM, Van der Noordaa J, Kruk MR, Meelis W, Van der
approaches to the study of behavior. Dordrecht: Kluwer,
Poel AM. Interactions between simultaneously activated beha-
vioural systems in the rat. Behav Neurosci 1989;103:784±789.
[102] Kemble ED, Thornton AE, Schultz LA. Some fear potentiating
[124] Leslie GB. Central stimulant properties of compounds with periph-
effects of ¯uprazine hydrochloride in mice. Aggress Behav
eral muscarinic properties. Nature 1965;208:1291±1293.
[125] Levinson PK, Flynn JP. The objects attacked by cats during stimula-
[103] Khantzian EJ. Opiate addiction: a critique of theory and some impli-
tion of the hypothalamus. Anim Behav 1965;13:217±220.
cations for treatment. Am J Psychother 1974;28:59±70.
[126] Leyhausen P. Cat behavior. The predatory and social behavior of
[104] Khantzian EJ. Psychological (structural) vulnerabilities and the
domestic and wild cats. New York: Garland STPM Press, 1979.
speci®c appeal of narcotics. Ann N Y Acad Sci 1982;398:24±32.
[127] Lion JR. Benzodiazepines in the treatment of aggressive patients. J
[105] King MB, Hoebel BG. Killing elicited by brain stimulation in rats.
Clin Psychiatry 1979;40:70±71.
Communications in Behavioral Biology 1968;2:173±177.
[128] Lorenz K. On aggression. London: Methuen, 1966.
[106] Kono R. Excitatory and inhibitory effects of acetylcholine (ACh) on
[129] Lu C-L, Shaikh MB, Siegel A. Role of NMDA receptors in hypotha-
the hypothalamic emotional behavior of the cat. Jpn J Pharmacol
lamic facilitation of feline defensive rage elicited from the midbrain
periaqueductal gray. Brain Res 1992;581:123±132.
[107] Kono R, Tashiro N, Nakao H. Inhibitory effects of acetylcholine on
[130] Luiten PGM, Koolhaas JM, de Boer S, Koopmans SJ. The cortico-
aggressive-defense reaction induced by electrical stimulation of the
medial amygdala in the central nervous system organization of
hypothalamus in cats. Brain Res Bull 1986;16:491±495.
agonistic behavior. Brain Res 1985;332:283±297.
[108] Koolhaas JM. Hypothalamically induced intraspeci®c aggressive
[131] Luo B, Cheu JW, Siegel A. Cholecystokinin B receptors in the
behaviour in the rat. Exp Brain Res 1978;32:365±375.
periaqueductal gray potentiate defensive rage behavior elicited
[109] Koolhaas JM, Van den Brink THC, Roozendaal B, Boorsma F.
from the medial hypothalamus of the cat. Brain Res (in press).
Medial amygdala and aggressive behavior: interaction between
[132] MacDonnell MF, Fessock L. Some effects of ethanol, amphetamine,
testosterone and vasopressin. Aggress Behav 1990;16:223±229.
disul®ram and p-CPA on seizing of prey in feline predatory attack
[110] Krettek JE, Price JL. Amygdaloid projections to subcortical struc-
and on associated motor pathways. Quart J Stud Alc 1972;33:437±
tures within the basal forebrain and brainstem in the rat and cat. J
Comp Neurol 1978;178:225±254.
[133] MacDonnell MF, Fessock L, Brown SH. Aggression and associated
[111] Krstic SK, Stefanovic-Denic K, Beleslin DB. Effect of morphine and
neural events in cats. Effects of p-chlorophenylalanine compared
morphine-like drugs on carbachol-induced ®ghting in cats. Pharma-
with alcohol. Quart J Stud Alc 1971;32:748±763.
col Biochem Behav 1982;17:371±373.
[134] Maeda H. Effects of psychotropic drugs upon the hypothalamic rage
[112] Kruk MR. Ethology and pharmacology of hypothalamic aggression
response in cats. Folia Psychiatrica et Neurologica Japonica
in the rat. Neurosci Biobehav Rev 1991;15:527±538.
[113] Kruk MR, Van der Laan CE, Meelis W, Phillips RE, Mos J, Van der
[135] Maeda H, Maki S. Dopaminergic facilitation of recovery from
Poel AM. Brain-stimulation induced agonistic behaviour: a novel
amygdaloid lesions which affect hypothalamic defensive attack in
paradigm in ethopharmacological aggression research. Prog Clin
cats. Brain Res 1986;363:135±140.
Biol Res 1984;167:157±177.
[136] Maeda H, Sato T, Maki S. Effects of dopamine agonists on hypotha-
[114] Kruk MR, Van der Laan CE, Mos J, Van der Poel AM, Meelis W,
lamic defensive attack in cats. Physiol Behav 1985;35:89±92.
Olivier B. Comparison of aggressive behaviour induced by electrical
[137] Malick JB. Effects of selected drugs on stimulus-bound emotional
stimulation in the hypothalamus of male and female rats. Prog Brain
behavior elicited by hypothalamic stimulation in the cat. Archives
Internationales De Pharmacodynamie et de Therapie 1970;186:137±
[115] Kruk MR, Van der Laan CE, Van der Spuy J, Van Erp AMM, Meelis
W. Strain differences in attack patterns elicited by electrical
[138] Marini JL, Walters JK, Sheard MH. Effects of d- and l-amphetamine
A. Siegel et al. / Neuroscience and Biobehavioral Reviews 23 (1999) 359±389
on hypothalamically-elicited movement and attack in the cat. Agres-
and Impulse Control, ACNP-Proceedings, Washington, DC.
[139] Mark VH, Takada I, Tsutsumi H, Takamatsu H, Toth E, Mark DB.
[159] Olivier B, Olivier-Aardema R, Wiepkema PR. Effect of anterior
Effect of exogenous catecholamines in the amygdala of a rage''
hypothalamic and mammillary area lesions on territorial aggressive
cat. Appl Neurophysiol 1975;38:61±72.
behavior in male rats. Behav Brain Res 1983;9:59±81.
[140] Masserman J. Is the hypothalamus a center of emotion? Psychosom
[160] Olivier B, van Dalen D. Social behavior in rats and mice: an etho-
Med 1941;3:3±25.
logical based model for differentiating psychoactive drugs. Aggress
[141] McCarty RC, Whitesides GH, Tomosky TK. Effects of p-chlorophe-
nylalanine on the predatory behavior of onychomys torridus. Phar-
[161] Panksepp J. Aggression elicited by electrical stimulation of the
macol Biochem Behav 1976;4:217±220.
hypothalamus in albino rats. Physiol Behav 1971;6:321±329.
[142] Miczek K, Haney M, Tidey J, Vivian J, Weerts E. Neurochemistry
[162] Panksepp J. Drugs and stimulus-bound attack. Physiol Behav
and pharmacotherapeutic management of aggression and violence.
In: Reiss A, Miczek K, Roth J, editors. Understanding and prevent-
[163] Panksepp J, Trowill J. Electrically induced affective attack from
ing violence, vol. 2, biobehavioral in¯uences. Washington, DC:
the hypothalamus of the albino rat. Psychon Sci 1969;16:118±
National Academy Press, 1994:245±514.
[143] Miczek KA, Donat P. Brain 5-HT system and inhibition of aggres-
[164] Penaloza-Rojas JH, Bach-Y-Rita G, Rubio-Chevannier HF, Hernan-
sive behaviour. In: Bevan P, Cools AR, Archer T, editors. Beha-
dez-Peon R. Effects of imipramine upon hypothalamic and amygda-
vioural pharmacology of 5-HT. Hove: Lawrence Erlbaum
loid excitability. Exp Neurol 1961;4:205±213.
[165] Pott CB, Kramer SZ, Siegel A. Central gray modulation of affective
[144] Middlemis DN. Stereoselective blockade at 3H5-HT binding sites
defense in differentially sensitive to naloxone. Physiol Behav
and at the 5-HT autoreceptor by propanolol. European Journal of
[166] Pucilowski O, Plaznik A, Kostowski W. Aggressive behavior inhibi-
[145] Middlemis DN, Blakeborough L, Leather SR. Direct evidence for an
tion by serotonin and quipazine injected into the amygdala in the rat.
interaction of b-adrenergic blockers with the 5-HT receptor. Nature
Behav Neural Biol 1985;43:58±68.
[167] Rao R, Yamano M, Shiosaka S, Shinohara A, Tohyama M. Origin of
[146] Mitchell PJ, Redfern PH. Potentiation of the time-dependent, anti-
leucine-enkephalin ®bers and their two main afferent pathways in
depressant-induced changes in the agonistic behaviour of resident
the bed nucleus of the stria terminalis in the rat. Exp Brain Res
rats by the 5-HT1A receptor antagonist, WAY-100635. Behavioural
[168] Rapoport JL. The biology of obsessions and compulsions. Sci Am
[147] Mos J, Kruk MR, Van der Poel AM, Meelis W. Aggressive behavior
induced by electrical stimulation in the midbrain central gray of
[169] Reis DJ, Fuxe K. Depletion of noradrenaline in brainstem neurons
male rats. Aggress Behav 1982;8:261±284.
during sham rage behaviour produced by acute brainstem transection
[148] Mos J, Lammers JHCM, Van der Poel AM, Bermond B, Meelis W,
in cat. Brain Res 1968;7:448±451.
Kruk MR. Effects of midbrain central gray lesions on spontaneous
[170] Reis DJ, Fuxe K. Brain norepinephrine: evidence that neuronal
and electrically induced aggression in the rat. Aggress Behav
release is essential for sham rage behavior following brainstem
transection in cat. Proc Natl Acad Sci USA 1969;64:108±112.
[149] Mos J, Olivier B, Lammers JHCM, Van der Poel AM, Kruk MR,
[171] Reis DJ, Miura M, Weinbren M, Gunne LM. Brain catecholamines:
Zethof T. Postpartum aggression in rats does not in¯uence threshold
relation to defense reaction evoked by acute brainstem transection in
currents for EBS-induced aggression. Brain Res 1987;404:263±266.
cat. Science 1967;156:1768±1776.
[150] Moss MS, Glazer EJ, Basbaum AI. The peptidergic organization of
[172] Roberts WW. Are hypothalamic motivational systems functionally
the cat periaqueductal gray. I. The distribution of immunoreactive
and anatomically speci®c? Brain Behav Evol 1969;2:317±342.
enkephalin-containing neurons and terminals. J Neurosci
[173] Roberts WW, Nagel J. First-order projections activated by stimula-
tion of hypothalamic sites eliciting attack and ¯ight in rats. Behav
[151] Moyer KE. Kinds of aggression and their physiological basis.
Communications in Behavioral Biology 1968;2:65±87.
[174] Roeling TAP, Kruk MR, Schuurmans R, Veening JG. Behavioural
[152] Nakao H, Tashiro N, Kono R, Araki R, Effects of GABA and glycine
responses of bicucculline methiodide injections into the ventral
on aggressive-defense reaction produced by electrical stimulation of
hypothalamus of freely moving, socially interacting rats. Brain Res
the ventromedial hypothalamus in cats. In: Itoh M, editor. Integra-
tive control functions of the brain. Amsterdam: Elsevier, 1979:332-
[175] Roeling TAP, Veening JG, Kruk MR, Peters JPW, Vermelis
MEJ, Nieuwenhuys R. Efferent connections of the hypothala-
[153] Nygren L-G, Olson L. A new major projection from locus coeruleus:
mic aggression area'' in the rat. Neuroscience 1994;59:
the main source of noradrenergic nerve terminals in the ventral and
dorsal columns of the spinal cord. Brain Res 1977;132:85±93.
[176] Roeling TAP, Veening JG, Peters JPW, Vermelis MEJ, Nieuwen-
[154] Olivier B. The ventromedial hypothalamus and aggressive beha-
huys R. Efferent connections of the hypothalamic grooming area''
viour in rats. Aggress Behav 1977;3:47±56.
in the rat. Neuroscience 1993;56:199±225.
[155] Olivier B. Selective anti-aggressive properties of DU 27725: etho-
[177] Romaniuk A, Brudzynski S, Gronska J. The effect of chemical
logical analyses of intermale and territorial aggression in the male
blockade of hypothalamic cholinergic system on defensive reactions
rat. Pharmacol Biochem Behav 1981;14(Suppl 1):61±77.
in cats. Acta Physiol Pol 1973;XXIV:809±816.
[156] Olivier B, Mos J. Maternal aggression in rats: effects of chlordiazep-
[178] Romaniuk A, Brudzynski S, Gronska J. The effects of intrahypotha-
oxide and ¯uprazine. Psychopharmacology (Berl) 1985;86:68±76.
lamic injections of cholinergic and adrenergic agents on defensive
[157] Olivier B, Mos J, Rasmussen DL. Behavioural pharmacology of the
behavior in cats. Acta Physiol Pol 1974;XXV:297±305.
serenic, eltoprazine. In: Raghoebar M, Olivier B, Rasmussen DL,
[179] Romaniuk A, Filipczak M, Fryczak J. The in¯uence of injection of
Mos J, editors. Drug metabolism and drug interactions. London:
5,6-dihydroxytryptamine to the dorsal raphe nucleus on carbachol-
Freund Publishing House, 1990:31±83.
induced defensive behavior and regional brain amine content in the
[158] Olivier B, Mos J, Tulp M, Schipper J, DenDaas S, Van Oortmerssen
cat. Pol J Pharmacol Pharm 1987;39:17±25.
G. Serotonergic involvement in aggressive behaviour in animals. In:
[180] Romaniuk A, Golebiewski H. Adrenergic modulation of the
Van Praag HM, editor. Monoaminergic Regulation of Aggression
hypothalamic cholinergic mechanism in the control of
A. Siegel et al. / Neuroscience and Biobehavioral Reviews 23 (1999) 359±389
emotional±defensive behavior in the cat. Acta Neurobiol Exp
[202] Siegel A, Edinger H. Neural control of aggression and rage. In:
Morgane PJ, Panksepp J, editors. Handbook of the hypothalamus.
[181] Roy A. Monoamines, glucose metabolism, suicidal and aggressive
New York: Marcel Dekker, 1981:203±40.
behaviors. Psychopharmacol Bull 1986;22:661±665.
[203] Siegel A, Edinger H, Dotto M. Effects of electrical stimulation of the
[182] Schubert K, Shaikh MB, Han Y, Pohorecky L, Siegel A. Differential
lateral aspect of the prefrontal cortex upon attack behavior in cats.
effects of ethanol on feline rage and predatory attack behavior: an
Brain Res 1975;93:473±484.
underlying neural mechanism. Alcohol Clin Exp Res 1996;20:882±
[204] Siegel A, Edinger H, Lowenthal H. Effects of electrical stimulation
of the medial aspect of the prefrontal cortex upon attack behavior in
[183] Schubert K, Shaikh MB, Siegel A. NMDA receptors in the midbrain
cats. Brain Res 1974;66:467±479.
periaqueductal gray mediate hypothalamically evoked hissing beha-
[205] Siegel A, Edinger HM. Role of the limbic system in hypothalami-
vior in the cat. Brain Res 1996;726:80±90.
cally elicited attack behavior. Neurosci Biobehav Rev 1983;7:395±
[184] Shaikh MB, Barrett JA, Siegel A. The pathways mediating affective
defense and quiet biting attack behavior from the midbrain central
[206] Siegel A, Pott CB. Neural substrate of aggression and ¯ight in the
gray of the cat: an autoradiographic study. Brain Res 1987;437:9±
cat. Prog Neurobiol 1988;31:261±283.
[207] Siegel A, Schubert KL, Shaikh MB. Neurotransmitters regulating
[185] Shaikh MB, Brutus M, Siegel A, Siegel HE. Topographically orga-
defensive rage behavior in the cat. Neurosci Biobehav Rev
nized midbrain modulation of predatory and defensive aggression in
the cat. Brain Res 1985;336:308±312.
[208] Siegel A, Skog D. Effects of electrical stimulation of the septum
[186] Shaikh MB, Brutus M, Siegel A, Siegel HE. Regulation of feline
upon attack behavior elicited from the hypothalamus in the cat.
aggression by the bed nucleus of stria terminalis. Brain Res Bull
Brain Res 1970;23:371±380.
[209] Siegel A, Troiano R, Edinger H. The pathway from the mediodorsal
[187] Shaikh MB, Brutus M, Siegel H, Siegel A. Differential control of
nucleus to the hypothalamus in the cat. Exp Neurol 1973;38:202±
aggression by the midbrain. Exp Neurol 1984;83:436±442.
[188] Shaikh MB, deLanerolle NC, Siegel A. Serotonin 5-HT1A and 5-HT2/
[210] Stoddard-Apter S, Levin B, Siegel A. A sympathoadrenal and cardi-
1C receptors in the midbrain periaqueductal gray differentially modu-
ovascular correlates of aggressive behavior in the awake cat. J Auton
late defensive rage behavior elicited from the medial hypothalamus
Nerv Syst 1983;8:343±360.
of the cat. Brain Res 1997;765:198±207.
[211] Stoddard-Apter SL, MacDonnell MF. Septal and amygdalar effer-
[189] Shaikh MB, Edinger H, Siegel A. Carbamazepine regulates feline
ents to the hypothalamus which facilitate hypothalamically elicited
aggression elicited from the midbrain periaqueductal gray. Pharma-
intraspeci®c aggression and associated hissing in the cat. An auto-
col Biochem Behav 1987;30:409±415.
radiographic study. Brain Res 1980;193:19±32.
[190] Shaikh MB, Lu C-L, MacGregor M, Siegel A. Dopaminergic regu-
[212] Sweidan S, Edinger H, Siegel A. The role of D1 and D2 receptors in
lation of quiet biting attack behavior in the cat. Brain Res Bull
dopamine agonist-induced modulation of affective defense behavior
in the cat. Physiol Biochem Behav 1990;36:491±499.
[191] Shaikh MB, Lu C-L, Siegel A. Affective defense behavior elicited
[213] Sweidan S, Edinger H, Siegel A. D2 dopamine receptor-mediated
from the feline midbrain periaqueductal gray is regulated by mu- and
mechanisms in the medial preoptic±anterior hypothalamus regulate
delta-opioid receptors. Brain Res 1991;557:344±348.
affective defense behavior in the cat. Brain Res 1991;549:127±
[192] Shaikh MB, Lu C-L, Siegel A. An enkephalinergic mechanism
involved in amygdaloid suppression of affective defence behavior
[214] Torda C. Effects of catecholamines on behavior. J Neurosci Res
elicited from the midbrain periaqueductal gray in the cat. Brain Res
[215] Tsumagari T, Nakajima A, Fukuda T, Shuto S, Kenjo T, Morimoto
[193] Shaikh MB, Schubert K, Siegel A. Basal amygdaloid facilitation of
Y, Takigawa Y. Pharmacological properties of 6-(o-chlorophenyl)-
midbrain periaqueductal gray elicited defensive rage behavior in the
cat is mediated through NMDA receptors. Brain Res 1994;635:187±
(Y-7131), a new anti-anxiety drug. Arzneimittelforschung
[194] Shaikh MB, Shaikh AB, Siegel A. Opioid peptides within the
[216] Uhl GR, Kuhar MJ, Synder SH. Enkephalin-containing pathway:
midbrain periaqueductal gray suppress affective defense behavior
amygdaloid efferents in the stria terminalis. Brain Res
in the cat. Peptides 1988;9:999±1004.
[195] Shaikh MB, Siegel A. Naloxone-induced modulation of feline
[217] Valenstein ES, Cox VC, Kakolewski JW. Reexamination of the role
aggression elicited from midbrain periaqueductal gray. Pharmacol
of the hypothalamus in motivation. Psychol Rev 1970;77:16±31.
Biochem Behav 1989;31:791±796.
[218] Van der Poel AM, Mos J, Kruk MR, Olivier B. A motivational
[196] Shaikh MB, Siegel A. GABA-mediated regulation of feline aggres-
analysis of ambivalent actions in the agonistic behaviour of rats in
sion elicited from midbrain periaqueductal gray upon affective
tests used to study effects of drugs on aggression. Prog Clin Biol Res
defense behavior in the cat. Brain Res 1990;507:51±56.
[197] Shaikh MB, Steinberg A, Siegel A. Evidence that substance P is
[219] Van der Poel AM, Olivier B, Mos J, Kruk MR, Meelis W, Van Aken
utilized in medial amygdaloid facilitation of defensive rage behavior
JHM. Anti-aggressive effect of a new phenylpiperazine compound
in the cat. Brain Res 1993;625:283±294.
(DU27716) on hypothalamically induced behavioural activities.
[198] Sheard MH. The effects of amphetamine on attack behavior in the
Pharmacol Biochem Behav 1982;17:147±153.
cat. Brain Res 1967;5:330±338.
[220] Van Erp AMM, Kruk MR, Meelis W, Veening JG. Periaqueductal
[199] Sheard MH. Clinical pharmacology of aggressive behavior. Clin
gray lesions do not affect grooming, induced electrically in the
hypothalamic paraventricular area in the rat. Behav Brain Res
[200] Siegel A. Aggression in epilepsy: animal models. In: Devinsky O,
Theodore WH, editors. Epilepsy and behavior. New York: Wiley/
[221] Varszegi MK, Decsi L. Some characteristics of the rage reaction
evoked by chemical stimulation of the hypothalamus. Acta Physio-
[201] Siegel A, Brutus M. Neural substrates of aggression and rage in the
logica Academiae Scientiarum Hungaricae 1967;32:61±68.
cat. In: Epstein AN, Morrison AR, editors. Progress in psychobiol-
[222] Vergnes M, Depaulis A, Boehrer A, Kempf E. Selective increase of
ogy and physiological psychology. San Diego, CA: Academic Press,
offensive behavior in the rat following intrahypothalamic 5,7-DHT-
induced serotonin depletion. Behav Brain Res 1988;29:85±91.
A. Siegel et al. / Neuroscience and Biobehavioral Reviews 23 (1999) 359±389
[223] Vergnes M, Karli P. DeÂclenchement d'un comportement d'agression
[229] Weiner S, Shaikh MB, Shaikh AB, Siegel A. Enkephalinergic invol-
par stimulation eÂlectrique de l'hypothalamus meÂdian chez le rat.
vement in periaqueductal gray control of hypothalamically elicited
Physiol Behav 1970;4:1427±1430.
predatory attack in the cat. Physiol Behav 1991;49:1099±1105.
[224] Vochteloo JD, Koolhaas JM. Medial amygdala lesions in male rats
[230] Wetherill GB. Sequential estimation of points on quantal response
reduce aggressive behavior: interference with experience. Physiol
curves. In: Barrie G, Wetherill GB, editors. Sequential methods in
statistics. London: Meuthen, 1966:162±79.
[225] Von Holst E, Von Saint Paul U. On the functional organization of
[231] Woodworth CH. Attack elicited in rats by electrical stimulation of
drives. Anim Behav 1963;11:1±20.
the lateral hypothalamus. Physiol Behav 1971;6:345±353.
[226] Waldbillig RJ. Attack, eating, drinking and gnawing elicited by
[232] Wurmser L. Psychoanalytic considerations of the etiology of
electrical stimulation of rat mesencephalon and pons. J Comp
compulsive drug use. J Am Psychoanal Assoc 1973;22:820±
Physiol Psychol 1975;89:200±212.
[227] Wasman M, Flynn JP. Directed attack elicited from hypothalamus.
[233] Yoshimura H, Kihara Y, Ogawa N. Psychotropic effects of adrener-
Arch Neurol 1962;6:220±227.
gic b-blockers on agonistic behavior between resident and intruder
[228] Watson RE, Troiano R, Poulakos JJ, Weiner S, Block CH, Siegel A.
mice. Psychopharmacology (Berl) 1989;91:445±50.
A [14C]2-deoxyglucose analysis of the functional neural pathways
[234] Zablocka B, Esplin D. Central excitatory and neuromuscular paral-
of the limbic forebrain in the rat. I. The amygdala. Brain Res Brain
yzant effects of pilocarpine in cats. Arch Int Pharmacodyn Ther
Res Rev 1983;5:1±44.
Source: https://newtimes.pl/wp-content/uploads/pdf/Neuropharmacology-of-brain-stimulation-evoked-aggression.pdf
Cobre Montana NL POWERING A CLEAN ENERGY FUTURE Presentation to the Annual General Meeting 24 November, 2014 For personal use only DISCLAIMER Cobre Montana NL This presentation is for information purposes only. Neither this presentation nor the information contained in it constitutes an offer, invitation, solicitation or recommendation in relation to the purchase or sale of shares in any jurisdiction. This presentation may not be distributed in any jurisdiction except in accordance with the legal requirements applicable in such jurisdiction. Recipients should inform themselves of the restrictions that apply in their own jurisdiction. A failure to do so may result in a violation of securities laws in such jurisdiction. This presentation does not constitute financial product advice and has been prepared without taking into account the recipients investment objectives, financial circumstances or particular needs and the opinions and recommendations in this presentation are not intended to represent recommendations to particular persons. Recipients should seek professional advice when deciding if an investment is appropriate. All securities transactions involve risks which include, amongst others, the risk of adverse or unanticipated market, financial or political developments. Certain statements contained in this presentation, including information as to the future financial or operating performance of Cobre Montana NL ("Cobre Montana" or "the Company") and its projects, are forward-looking statements. Such forward-looking statements are necessarily based upon a number of estimates and assumptions that, whilst considered reasonable by Cobre Montana, are inherently subject to significant technical, business, economic, competitive, political and social uncertainties and contingencies; involve known and unknown risks and uncertainties that could cause actual events or results to differ materially from estimated or anticipated events or results reflected in such forward-looking statements; and may include, among other things, statements regarding targets, estimates and assumptions in respect of potash and phosphate production and prices, operating costs and results, capital expenditures, ore reserves and mineral resources and anticipated grades and recovery rates, and are or may be based on assumptions and estimates related to future technical, economic, market, political, social and other conditions. Cobre Montana disclaims any intent or obligation to update publicly any forward-looking statements, whether as a result of new information, future events or results or otherwise. The words "believe", "expect", "anticipate", "indicate", "contemplate", "target", "plan", "intends", "continue", "budget", "estimate", "may", "will", "schedule" and other similar expressions identify forward-looking statements. All forward-looking statements made in this presentation are qualified by the foregoing cautionary statements. Investors are cautioned that forward looking statements are not guarantees of future performance and accordingly investors are cautioned not to put undue reliance on forward-looking statements due to the inherent uncertainty therein. Many known and unknown factors could cause actual events or results to differ materially from estimated or anticipated events or results reflected in such forward-looking statements. Such factors include, but are not limited to: competition; mineral prices; ability to meet additional funding requirements; exploration, development and operating risks; uninsurable risks; uncertainties inherent in ore reserve and resource estimates; dependence on third party smelting facilities; factors associated with foreign operations and related regulatory risks; environmental regulation and liability; currency risks; effects of inflation on results of operations; factors relating to title to properties; native title and aboriginal heritage issues; dependence on key personnel; and share price volatility and also include unanticipated and unusual events, many of which are beyond the Company's ability to control or predict. COMPETENT PERSON'S STATEMENT The information in this report that relates to reporting of Exploration Results based on and fairly represents information and supporting documentation prepared by Adrian Griffin, who is a member of
La plongée souterraine est un loisir sportif en pleine expansion, souvent médiatisée lors d'incidents spectaculaires. Des travaux récents (0) ont permis de constater que parmi le nombre d'accidents mortels survenus lors de plongées souterraines en France, certains décès succèdent à des affections médicales préexistantes. Afin de poursuivre la prévention des accidents en plongée souterraine, nous nous proposons