Space.geocities.jp
Ligand binding and co-activator
assembly of the peroxisome
proliferator-activated receptor-g
Robert T. Nolte*†, G. Bruce Wisely*†, Stefan Westin‡, Jeffery E. Cobb§, Millard H. Lambert*, Riki Kurokawa‡,Michael G. Rosenfeldk, Timothy M. Willson§, Christopher K. Glass‡ & Michael V. Milburn*
*
Department of Structural Chemistry, and §
Department of Medicinal Chemistry, Division of Chemistry, Glaxo Wellcome Research and Development,Research Triangle Park, North Carolina 27709, USA‡
Division of Cellular and Molecular Medicine, and k
Howard Hughes Medical Institute, Department of Medicine, University of California, San Diego, La Jolla,California 92093-0651, USA†
These authors contributed equally to this work
The peroxisome proliferator-activated receptor-g
(PPAR-g
) is a ligand-dependent transcription factor that is important
in adipocyte differentiation and glucose homeostasis and which depends on interactions with co-activators, including
steroid receptor co-activating factor-1 (SRC-1). Here we present the X-ray crystal structure of the human apo-PPAR-g
ligand-binding domain (LBD), at 2.2 A˚ resolution; this structure reveals a large binding pocket, which may explain the
diversity of ligands for PPAR-g
. We also describe the ternary complex containing the PPAR-g
LBD, the antidiabetic
ligand rosiglitazone (BRL49653), and 88 amino acids of human SRC-1 at 2.3 A˚ resolution. Glutamate and lysine
residues that are highly conserved in LBDs of nuclear receptors form a ‘charge clamp' that contacts backbone atoms of
the LXXLL helices of SRC-1. These results, together with the observation that two consecutive LXXLL motifs of SRC-1
make identical contacts with both subunits of a PPAR-g
homodimer, suggest a general mechanism for the assembly of
nuclear receptors with co-activators.
The peroxisome proliferator-activated receptor belongs to the
receptor structures is folded against the LBD and the AF-2 hydro-
family of nuclear receptors that includes the oestrogen receptors,
phobic residues form part of the ligand-binding pocket. On the
thyroid hormone receptors, retinoic-acid receptors (RARs) and
basis of these observations, a ‘mouse trap' model of receptor
retinoid-X receptors (RXRs)1. Although many of these receptors
activation has been proposed, in which the AF-2 helix closes on
have well-studied physiological ligands, such as steroids, thyroxine
the ligand-binding site in response to ligand and establishes a
and retinoids, more than half of the gene family are ‘orphan'
transcriptionally active form of the receptor18.
receptors that, in many cases, are important in development and
There is evidence that nuclear receptors require the ligand-
gene regulation but have not yet been associated with a physiolo-
dependent recruitment of co-activator proteins to effectively sti-
gically relevant ligand. A
bona fide cellular ligand for PPAR has not
mulate gene transcription, which is dependent on allosteric altera-
yet been found; however, several essential fatty acids, oxidized lipids
tions in the AF-2 helical domain20,21. Indeed, all known ligand-
and prostaglandin J derivatives can bind and activate the receptor2,3.
dependent nuclear receptors require the AF-2 domain for effective
The three known human PPAR subtypes, a, g and d, show
interaction with co-activators22–25. Among the best studied of the
distinct tissue distributions4,5 and are associated with selective
co-activators are the p160 proteins, which were initially identified
ligands3,4,6. PPAR-g is expressed at high levels in adipose tissue
biochemically as ligand-dependent nuclear-receptor-interacting
and macrophages and can strongly induce adipogenesis of preadi-
factors and whose genes were cloned and identified as members
pocyte cells, indicating that an endogenous PPAR-g ligand may be
of a gene family of steroid receptor co-activating factors, referred to
an important regulator of adipogenesis5,7–12. At submicromolar
as SRC-1/NcoA-1, TIF2/GRIP-1/NcoA-2 and pCIP/ACTR/AIB1
levels, a class of compounds known as thiazolidinediones (TZDs)
(refs 26–32). The nuclear-receptor-interaction domain of these
activates PPAR-g and are used pharmaceutically for the treatment
factors is highly conserved and contains three repeated motifs of
of type 2 diabetes because they sensitize tissues to insulin13,14. PPAR-
the consensus sequence LXXLL (where X is any amino acid), each of
g may be the biochemical target of TZDs6,13.
which is sufficient for ligand-dependent interaction with several
PPAR-g contains the general structural features of other nuclear-
receptor-family members, including a central DNA-binding
Here we report X-ray structure analysis of the apo-PPAR-g LBD,
domain and a carboxy-terminal domain that mediates ligand-
and of the ternary structure consisting of the PPAR-g LBD, the
binding, dimerization and transactivation functions. PPAR-g
antidiabetic ligand rosiglitazone35, and a region of the SRC-1
must form a heterodimer with RXR to bind DNA and activate
nuclear-receptor-interaction domain containing two LXXLL
transcription. Ligand-dependent transcription requires a highly
conserved motif, termed activating function-2 (AF-2), located atthe far C terminus of the LBD15. The structures of the LBDs of RXR,
Overall structure and refinement
RAR, the thyroid hormone receptor and the oestrogen receptor are
The results of the crystallographic refinement are summarized in
similar16–19. The LBDs are folded into three layers of a-helices that
Table 1. In both the apo and the ternary complex crystal structures,
allow the formation of a ligand-binding site, which is almost entirely
amino acids 207–476 were modelled into the electron-density map
buried within the core of these a-helices. In the structure of the
and crystallographically refined. The nomenclature of the second-
unliganded RXR, the AF-2 motif adopts an a-helical structure that
ary-structure elements (Fig. 1a) is based on the RXR LBD crystal
extends away from the LBD. In contrast, the AF-2 helix in the
structure16. Most PPAR-g amino acids were well defined in the
agonist-bound RAR, thyroid hormone receptor and oestrogen
electron-density map, except for the loop between helices H29 and
Nature Macmillan Publishers Ltd 1998
NATURE VOL 395 10 SEPTEMBER 1998
H3, which is the most thermally mobile loop. In the ternary
interfaces are conserved in both PPAR and RXR, namely, F432,
complex, SRC-1 was fitted to the electron density and crystal-
A433, L436, E418, E407 and K438 of PPAR-g.
lographically refined for amino acids 628–640 and 684–703. Forboth the apo and the ternary complex crystals, the same non-
crystallographic dimer configuration of PPAR-g is observed as in
The apo-PPAR-g ligand-binding site is a large T-shaped cavity that
the RXR-a and oestrogen receptor-a crystal structures16,19.
extends from the C-terminal helix to the b-sheet lying between
The crystal structure of the PPAR-g LBD reveals a bundle of 13 a-
helices H3 and H6. This domain is mainly hydrophobic and is
helices and a small four-stranded b-sheet (Fig. 1a). The PPAR-g
buried within the bottom half of the LBD (Fig. 1b). One region of
LBD is very similar to the overall fold of other nuclear-receptor
the domain is 20 A˚ in length, lies between H3 and the b-sheet, and is
structures from helix 3 to the C terminus. However, PPAR-
parallel to H3. Another cavity, which extends from the
unique in its overall tertiary structure and contains an extra helix,
the C-terminal AF-2 helix and is 16 A˚ in length, is orthogonal to the
designated H29, between the first b-strand and H3. The tertiary
T-shaped cavity, behind H3. The total volume of this domain in the
placement of H2 is different in PPAR-g compared with other
apo-PPAR-g crystal structure is ,1,300 A˚3 (Fig. 1b). A proposed
nuclear-receptor LBD tertiary structures, and provides easier
ligand-entry site in this cavity is apparent from the crystal structure
access for ligands. Helices H4, H5 and H8 are tightly packed
and lies between H3 and the b-sheet. The surface around the entry
between helices H1, H3, H7 and H10 at the top half of the LBD
site comprises several hydrophilic sidechain amino acids, namely,
(Fig. 1). This structural arrangement seems to be important in
D243, E290, R288 and E295. PPAR-g is probably unique among
forming the ligand-binding site: helices H3, H7 and H10 are
other nuclear-receptor LBDs in having this ligand-accessible
anchored to allow them to jut downwards and form the scaffolding
for the large cavity of the ligand-binding site in PPAR-g.
Rosiglitazone occupies roughly 40% of the ligand-binding site in
The crystal structures of the apo and ternary complexes of PPAR-
the ternary complex. In general the ligand is in a U-shaped
g contain a homodimer of the protein that involves a total surface conformation, wrapping around H3 with its central benzene ringarea of ,1,600 A˚2. Helix 10 of one monomer forms a coiled-coil
directly behind H3 and the TZD head group and pyridine ring
interaction with helix 10 of the other monomer, with amino acids
wrapping around H3 (Fig. 2). The TZD head group makes several
F432, A433, L436 and T440 of each monomer contributing to the
specific interactions with amino acids in H3, H4, H10 and the AF-2
interaction. Helices 9 and 10 and a loop before helix 9 contribute
helix (Fig. 2). The carbonyl groups of the TZD form hydrogen
several salt bridges to the dimer interface; these bridges are formed
bonds with two histidine residues, H323 and H449. Y473 in the AF-
between residues E418 and K422, E407 and K434, and K438 and
2 helix forms a secondary hydrogen bond with H323. The partly
D396. There is no evidence that PPAR-g functions as a homodimer
negatively charged nitrogen of the TZD head group is within
in vivo; however, both oestrogen receptor-a and RXR-a use the
hydrogen-bonding distance of the Y473 sidechain hydroxyl group.
same homodimer interface as that seen in PPAR-g. The similarity of
A buried lysine residue, K367, forms another secondary hydrogen
the homodimer interfaces in RXR and PPAR indicates that the
bond with the ligand, at residue H449. All of these primary and
PPAR and RXR homodimer interface may constitute the hetero-
secondary hydrogen bonds result in a fixed conformation of the
dimer PPAR–RXR interface for the LBDs. In fact, PPAR and RXR
TZD head group and of the participating amino acids.
share only 27% sequence identity within their LBDs, but several
Next to the head group, the sulphur atom of the TZD ring is
amino acids that form the strongest interactions at the homodimer
positioned in a hydrophobic region of the PPAR-g ligand-binding
Table 1 PPAR-g LBD structure determinations
Unique (
N)
SRC-1, rosiglitazone, PPAR-g
.
Space group
a ¼ 91:87 ˚A,
b ¼ 62:0 ˚A,
c ¼ 118:3 ˚A
a ¼ 52:21 ˚A,
b ¼ 69:06 ˚A,
c ¼ 177:97 ˚A
Unique (
N)
85% (60.0 A˚–2.2 A˚)
89.7% (20.0 A˚–2.3 A˚)
68% (2.3 A˚–2.2 A˚)
53.5% (2.4 A˚–2.3 A˚)
Rfree (%)(1 j)
r.m.s.d. bond lengths (A˚)
r.m.s.d. bond angles (degrees)
sym ¼ Sj
Iavg
† Phasing power ¼ Sj
F
scale ¼ Sj
FP
factor ¼ Sj
FP
Pcalc j=S
Fp
p and
FPcalc are observed and calculated structure factors,
Rfree is calculated for a randomly chosen 5% of reflections and
Rfactor is calculated for the
remaining 95% of reflections.
Selenomethionyl, a selenomethionine-incorporated protein; r.m.s.d., root mean square deviation from ideal geometry; %
N, percentage of observed reflections used in the crystallographicrefinement relative to the total theoretically possible. Apo native1 and native2 are two apo native data sets that were used for MIR structure detemrination and crystallographic refinement,respectively.
Nature Macmillan Publishers Ltd 1998
NATURE VOL 395 10 SEPTEMBER 1998
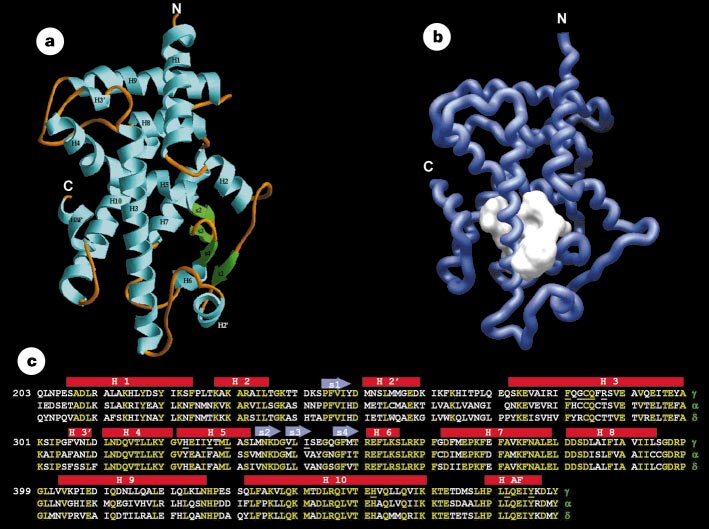
pocket formed by F363, Q286, F282 and L469. The central benzene
zone. The initial purification and crystallization of the co-expressed
ring of the ligand occupies a very narrow pocket between C285 and
PPAR-g and SRC-1 protein resulted in a molar ratio of 2:1 which
M364. The bridging oxygen atom between the benzene ring and the
was consistent with the observed solution molecular weight (see
pyridine ring provides vital geometry for the pyridine ring, which
Methods). The crystal structure shows that the 88 amino acids of
occupies the pocket between H3 and the b-sheet. Rosiglitazone has
SRC-1 bind to a homodimer of PPAR-g in the presence of ligand. In
one chiral atom in the head group that is in the S configuration, even
the ternary complex, one LXXLL motif binds to one molecule of
though a racemic mixture was used for crystallization.
PPAR-g and the second LXXLL motif binds to the other molecule of
a-Substituted carboxylic acids can act as bio-isosteric replace- PPAR-g, with the connecting protein spanning the homodimer
ments for the TZD head group, maintaining high affinity binding
and receptor activation36. We predict that these carboxylic acids
Nuclear-receptor AF-2 domains often contain a highly
conserve the key interactions with H323 and H449, and that this
glutamate residue which is important for transactivation but is not
molecular recognition may be important for the binding of other
required for ligand binding38,39. For example, mutation of this
acidic ligands. Several naturally occurring carboxylic acids, includ-
residue in RAR generates a dominant-negative receptor that is
ing essential fatty acids, oxidized lipids and prostaglandin J2
transcriptionally silent and can no longer bind to co-activator
metabolites, can bind and activate PPAR-g at micromolar
proteins39. Here, in the ternary complex, the side chain of this
concentrations2. We propose that all of these hydrophobic car-
residue, E471, forms hydrogen bonds with the backbone amides of
boxylic acids bind to PPAR, and that the key interactions involving
K632 and L633 in helical domain HD1 of the LXXLL motif and with
the histidine residues required for TZD binding are conserved with
the backbone amides of K688, I689 and L690 in helix HD2. In both
the acid groups. The other hydrophobic parts of the ligands would
HD1 and HD2, there is a lysine residue at the amino terminus of the
be free to interact in a relatively nonspecific manner with the large
amphiphatic sequence that is solvent-exposed in the crystal
ligand-binding pocket, resulting in the observed promiscuity of
structure and does not interact directly with the nuclear receptor.
PPAR. In effect, the extra helix, H29, and the altered tertiary
The C-terminal ends of HD motifs interact with the PPAR-g LBD by
placement of the H2 helix define PPAR as a nuclear receptor with
a similar hydrogen-bonding network to that seen at the N-terminal
a new hormone specificity; it has evolved to bind to multiple natural
ends. A highly conserved lysine, K301, is solvent-exposed in the
ligands with moderate affinity.
apo-PPAR-g structure and seems not to be involved in the folding ofthe LBD. In the ternary complex, the lysine side chain forms
Determinants of SRC-1 LXXLL interactions
hydrogen bonds with two backbone carbonyls of L636 and T639
Biochemical studies indicated that two LXXLL motifs were
in HD1 and L693 and L694 in HD2. There is also biochemical
required for cooperative interaction of SRC-1 with nuclear-
evidence that this lysine residue is important in transcriptional
receptor heterodimers37, and we chose to co-crystallize the PPAR-
activity and co-activator binding40. We propose that E471 and K301
g LBD with a region of SRC-1 containing LXXLL motifs 1 and 2 define a ‘charge clamp' that allows the orientation and placement of(SRC-1623–710) in the presence of the high-affinity ligand rosiglita-
the HD motif into the coactivator-binding site (Fig. 3).
Figure 1 Crystal structure of the apo-PPAR-g. a, Ribbons drawing of the apo-
between PPARs a, g and d are in yellow; the secondary structure that the
PPAR-g LBD, amino acids 207–476. The nomenclature of the helices is based on
sequence adopts in PPAR-g is shown in red boxes for a-helices and blue
the RXR-a crystal structure16. a-Helices are light blue; b-strands are green; loops
arrows for b-strands. Residues involved in rosiglitazone binding are underlined.
are brown. b, A worm backbone tracing of PPAR-g, with a surface representation
The sequence alignment predicts that several residues involved in direct inter-
of the unoccupied van der Waals space in the ligand-binding site. We determined
actions of PPAR-g with ligand are not conserved in the other PPAR subtypes, and
the ‘unoccupied' volume by fitting in virtual atoms that did not occupy the van der
explains the specificity of TZDs for PPAR-g: residue H323 is not found in PPAR-a,
Waals surface of the protein. The total unoccupied volume is ,1,300 A˚3. c,
and Q286 is not found in PPAR-a or PPAR-d.
Sequence alignment of the human PPAR LBDs. Amino acids that are conserved
Nature Macmillan Publishers Ltd 1998
NATURE VOL 395 10 SEPTEMBER 1998
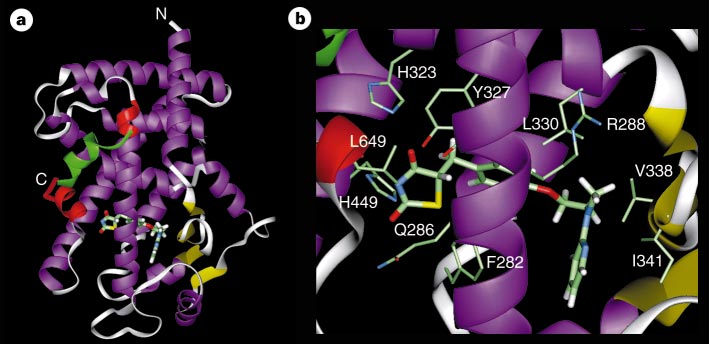
The hydrophobic face of the LXXLL helix of SRC-1 packs into a
and the threonine in HD1 or the glutamine in HD2. Although the
hydrophobic pocket formed between E471 and K301 of PPAR-g.
+6 residue may be important as a capping residue for helix
This pocket is formed from helix-packing interfaces of H3, H4, H5
stabilization, the side chain does not contact the surface of the
and the AF-2 helix (Fig. 3). Amino acids L468, L318, T297, Q314,
LBD and is unlikely to convey specificity to the HD. The +7 to +10
L311, V315, K301 and E471 of PPAR-g make up most of the binding
residues form no specific sequence, but these residues might interact
site for the interacting motifs of SRC-1. The total surface area of the
with other LBD domains and contribute to nuclear-receptor
region of interaction between LXXLL and PPAR-g is ,800 A˚2 and
involves specific salt bridges between the charge clamp on theoutside of the binding site and a buried hydrophobic core of the
Structure and function of AF-2 domains
LXXLL motif; L633, L636 and L690, I693 of the two SRC-1 motifs
g molecules in the ternary complex have nearly
interact hydrophobically with L468 and L318 of the PPAR-g LBD.
identical Ca conformations, with a root mean square (r.m.s.)
The ternary complex reveals some of the specificity determinants
deviation of 0.76 A˚. Although the two molecules in the apo-
that target the individual HD motifs of SRC-1 to specific nuclear
PPAR-g homodimer are very similar from the N terminus to
receptors. The charge clamp is presumably not selective in placing
amino acid 455, they exhibit entirely different conformations of
the HD motif into this site, although a particular nuclear-receptor
the AF-2 helix and the preceding loop. In one of the apo-PPAR-g
dimer may be selective for different co-activators. Selectivity is more
molecules, the AF-2 helix is folded against the LBD in a conforma-
likely to reside in the amino acids located N-terminally and C-
tion that is very similar to that in the ternary ligand-bound
terminally to the HD motif that are not conserved between co-
complex. In contrast, in the other molecule of the apo-PPAR-g
activators but which do interact with the PPAR-g LBD. A study of
homodimer the AF-2 helix adopts an extended conformation,
the individual contacts that the HD motifs make with the LBD
projecting slightly away from the LBD. Perhaps, in the apo receptor,
reveals the role that each residue may play in the recognition
the AF-2 helix can assume both an ‘active' (AF-2 helix in the
process. The residues at positions −3 and −2 of the HD motifs
liganded state) and an ‘inactive' conformation, with the ligand
(Table 2) do not appear to make any significant interactions with
acting to lock the receptor into the active conformation. This
the LBD; these residues rise up over the charge-clamp residue
scenario would be analogous to the proposed ‘mouse trap' model
E471 and away from the surface of the LBD. The amino acid at
of receptor activation18. However, a different explanation was
position −1 fits in a shallow pocket created by P467 and L468 of
suggested by analysis of the interaction of the SRC-1 HD with the
the AF-2 helix and the +4 residue of the HD motif. There is a
ligand-bound receptor. Closer examination of the apo-PPAR-g
preference for large hydrophobic residues at position −1 of the
structure revealed that the extended AF-2 helix contacted the
HD motif to allow optimal interaction with PPAR-g and, in the
charge clamp of a crystallographically related PPAR-g molecule in
crystal structure, a lysine residue interacts hydrophobically at this
a different homodimer (Fig. 3f). This crystal packing interaction
places the AF-2 helix of one PPAR-g molecule in an ‘inactive'
The residues at positions +2 and +3 of the HD motif face out into
conformation that allows it to bind to the AF-2 helix of another apo-
solution, making no sidechain contacts with the LBD, consistent
PPAR-g molecule in a crystallographically related homodimer that
with the lack of sequence conservation for these positions. The +4
is in a conformation similar to that observed for the liganded
residue lies in a hydrophobic pocket at the surface of the LBD. This
receptor. E471 and K301 of the ‘active' AF-2 helix form hydrogen
leaves the top of the pocket exposed to solvent, perhaps allowing it
bonds with the crystallographically related ‘inactive' AF-2 helix. The
to easily accommodate residues other than a leucine at this position.
potential generality of this arrangement of AF-2 domains is
The PPAR-g pocket that encloses the residue at position +5 of the
strengthened by analysis of the apo-RXR-a LBD crystal structure,
HD motif is built mainly from residues T297, A300, K301, F306,
in which the extended RXR-a AF-2 domain binds the LXXLL-
L311 and L318. Large hydrophobic residues, such as methionine or
binding pocket of a crystallographically related RXR molecule16.
phenylalanine, could fit into this pocket but would require a modestrearrangement of one of the surrounding groups or a shift of the +5
Mechanism of ligand activation
Ca position. The residues at the +6 position seem to form a
Ligand-dependent transcriptional activation by nuclear receptors
hydrogen bond between the main-chain carbonyl of the +2 residue
probably requires the recruitment of co-activator proteins such as
Figure 2 Rosiglitazone binding with the PPAR-g LBD and SRC-1 in the ternary
network of polar interactions that include the AF-2 domain. b, The secondary-
complex. a, Ribbons drawing showing the ternary complex of PPAR-g LBD,
structure elements are shown as a ribbon drawing, with amino acids involved in
BRL49653, and the LXXLL helix domain of SRC-1. Residues around K301 and E471
ligand binding labelled. Each atom is coloured separately with the same colour
that form the ‘charged clamp' are red, and the LXXLL SRC-1 helix is green.
scheme: light green, carbon; red, oxygen; blue, nitrogen; yellow, sulphur.
Rosiglitazone (stick diagram) binds in a deep cavity of the protein and provides a
Nature Macmillan Publishers Ltd 1998
NATURE VOL 395 10 SEPTEMBER 1998
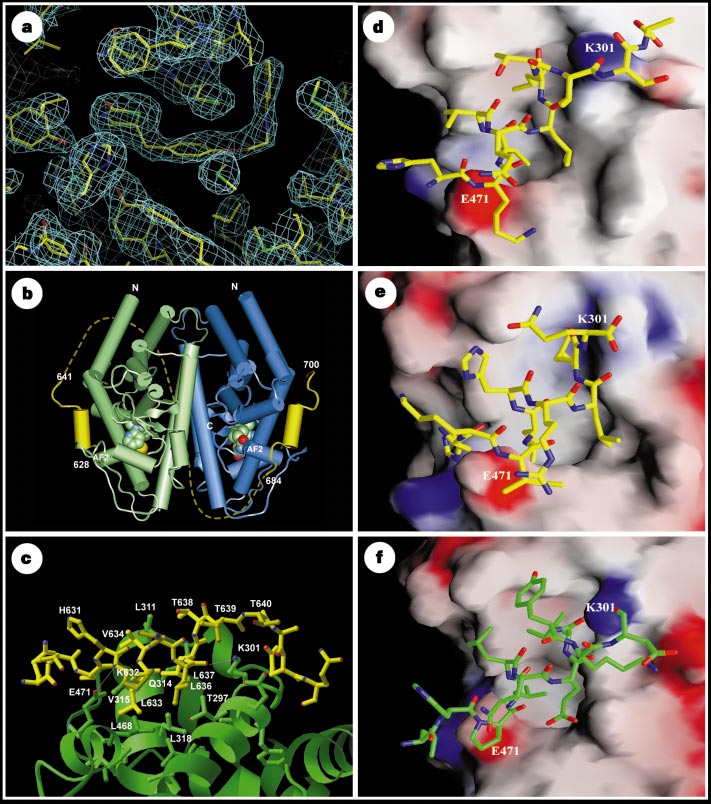
SRC-1 (refs 26–28, 33). Most co-activator proteins contain one or
and in AF-1 domains, indicating that this motif may play a general
more copies of the LXXLL motif. The ternary structure of PPAR-g
role in mediating interactions between components of cellular
shows that each member of the receptor dimer interacts with a
single LXXLL motif of SRC-1. The length and orientation of this
The observation that two HD motifs of SRC-1 make simulta-
helical motif is vital for proper backbone interactions with E471 in
neous contacts with both LBDs of the PPAR-g homodimer indicates
the receptor AF-2 domain and with K301 in helix H3 of the LBD.
that the multiple LXXLL motifs within co-activators may mediate
These residues, which are highly conserved among members of the
cooperative binding to nuclear-receptor dimers. In SRC-1, 52
nuclear-receptor family, define a ligand-dependent charge clamp
amino acids separate HD1 and HD2 and 54 amino acids separate
that positions the HD motif of LXXLL to allow the leucine residues
HD2 and HD3. There is a similar number of intervening amino
to pack tightly into a hydrophobic pocket in the receptor LBD. The
acids between the HDs in pCIP and TIF-2. The distance
residues that define this hydrophobic pocket are also highly con-
HD motifs may add more specificity to interactions with nuclear-
served among other members of the nuclear-receptor family,
receptor dimers. Although only two HD motifs would be necessary
suggesting a common mechanism for co-activator binding. The
for cooperative binding of SRC-1 to a nuclear-receptor dimer,
LXXLL motif is also present in several other transcription factors
perhaps the extra motif (HD3) may allow combinatorial
Figure 3 SRC-1 interactions with PPAR-g. a, A sigma-weighted 2Fo–Fc omit
backbone of the PPAR-g LBD is in green. PPAR-g amino acids binding to the
electron-density map is shown contoured at 1.0 j for the area surrounding the
LXXLL helix are also shown in green. d, Residues H631–T640 of SRC-1 are
rosiglitazone ligand. b, A ribbons drawing of the PPAR-g LBD dimer and SRC-1,
coloured as in c, with an electrostatic surface of PPAR-g showing the coactivator-
including the ligand rosiglitazone. The two PPAR-g monomers are blue and green
binding site. E471 and K301 side chains result in the red (negative) and blue
and the two SRC-1 interacting helices are yellow. The structure of SRC-1 was
(positive) charges on the surface of the coactivator-binding site at the N and C
determined from amino acids 628–640 and 684–703 and was crystallographically
termini of the SRC-1 helix, respectively. e, Residues H687–E696 of SRC-1 are
refined. Very weak electron density from residues 670 to 684 was visible but was
coloured as in c, with an electrostatic surface of PPAR-g showing the coactivator-
not crystallographically refined and is shown as a dashed line. SRC-1 amino acids
binding site. f, Amino acids L465–K474 of the PPAR-g AF-2 helix of one monomer
642–669 were disordered and not structurally determined. The diagram shows
in the apo structure are shown in: green, carbon; blue, nitrogen; red, oxygen, with
how one SRC-1 molecule, with two interacting domains, forms a complex with a
an electrostatic surface of PPAR-g showing the coactivator-binding site. E471 and
PPAR-g homodimer. The dashed line connecting the two structurally determined
K301 side chains result in the red (negative) and blue (positive) charges on the
domains of SRC-1 is the proposed connection between these two domains. c,
surface at the N and C terminus of the other PPAR-g monomer. This figure shows
The binding of SRC-1 (amino acids 628–642) to the LXXLL-binding site of PPAR-g.
how one monomer in the apo crystal structure orientates its AF-2 helix into the
SRC-1 is coloured: yellow, carbon; blue, nitrogen; red, oxygen. The ribbon
coactivator-binding site of another crystallographically related monomer.
Nature Macmillan Publishers Ltd 1998
NATURE VOL 395 10 SEPTEMBER 1998
Table 2 AF-2 and HD helix alignments
Subcloning and protein purification. Amino acids 176–477 were expressed
by subcloning into the T7 Escherichia coli expression vector, pRSETa
(Invitrogen). The His-tagged plasmid was transformed into BL21(DE3) cells,
which were grown at 25 8C, induced with 1 mM isopropyl-b-D-thiogalactoside
(IPTG) at A
¼ 1:0 for 1 h, and then collected. The His-tagged PPAR-g LBD
protein was purified with an affinity column of Ni2+-coupled Sepharose
(50 mM Tris-HCl, pH 8.0, 250 mM NaCl, 25 mM imidiazole, pH 7.5). The
protein was then subjected to limited proteolysis with Arg-C at 4 8C overnight 8
and then purified with a mono-Q–Sepharose column using a salt gradient. The
protein was N-terminal sequenced to ensure purity of .95% of the digested
Thyroid receptor-a
PPAR-g LBD amino acids 183–477. The selenomethionyl protein was prepared
in the same way as the wild-type protein.
Oestrogen receptor-a
The SRC1–PPARg complex was formed by expression in E. coli BL21(DE3)
Glucocorticoid receptor
of PPAR-g amino acids 206–477 (plasmid PRSETa; N-terminal His-tag
Progesterone receptor
MKKGHHHHHHG) and SRC-1 amino acids 623–710 (plasmid pACYC184;
Nuclear-receptor (NR) AF-2 helix motifs and co-activator helix motifs are aligned on the basis
N-terminal MKK). The transformed cells were grown at 22 8C, induced with
of the predicted helical structure overlap. The core box of hydrophobic residues that coulddirectly bind to the LXXLL-binding site of the LBD is underlined. There is a high degree of
1 mM IPTG at A
¼ 1:0 for 1 h, and then collected. The SRC1–PPARg
conservation in all of the HD1, HD2 and HD3 boxes, indicating a common function for the
complex was purified with an affinity column of Ni2+-coupled Sepharose
serial structure of these domains. The numbering for LXXLL motifs used in the text is shownat the top.
(50 mM Tris-HCl, pH 8.0, 250 mM NaCl, 25 mM imidiazole, pH 7.5). Twopeaks were isolated from the superdex-75 column; these peaks containedPPAR-g alone and SRC1–PPARg at a 2:1 molar ratio as quantified using N-terminal sequencing and amino-acid-composition analysis. The 2:1 molar ratio
interactions of the LXXLL motifs that are optimal for different
was also consistent with the observed solution molecular weight from gel-
receptor dimers.
filtration analysis and light-scattering measurements.
The observation that the AF-2 helix of an apo receptor can
Crystallization and data collection. The Arg-C-digested PPAR-g LBD was
interact with the LBD of a second receptor raises several questions
concentrated to 15 mg ml−1 (20 mM Tris, pH 8.0, 5 mM dithiothreitol (DTT),
regarding allosteric interactions between nuclear receptors and the
100 mM NaCl, 2 mM EDTA) and crystallized using the vapour phase diffusion
mechanisms of ligand-dependent activation. Although the crystal
method by adding equal volume amounts of concentrated protein and a
structures of the apo-PPAR-g and apo-RXR-a LBDs indicate that
crystallization buffer of 1.4 M sodium citrate and 100 mM HEPES (pH 7.5). We
the AF-2 domains contact the LXXLL-binding pockets of adjacent
added rosiglitazone to the SRC1–PPARg complex at a molar ratio of 5:1,
dimers, molecular modelling indicates that rotation around one
concentrated the complex to 10 mg ml−1 (25 mM Tris, pH 8.0, 5 mM DTT,
amino acid of RXR-a and extension of two amino acids at this
500 mM ammonium acetate) and crystallized it using the vapour phase
position would allow the RXR-a AF-2 domain to bind to the
diffusion method by adding equal volumes of concentrated protein and a
LXXLL-binding pocket of a dimeric partner. Specific partners of
crystallization buffer of 17% PEG 4000, 200 mM ammonium acetate and
RXR in heterodimers, including RAR and the thyroid hormone
20 mM HEPES (pH 7.5). Complete diffraction data were measured at −170 8C
receptor, allosterically inhibit the binding of ligands to RXR41,42.
with an R-axis II image plate system and an R-axis IV image plate system for the
Allosteric inhibition could be due, in part, to the interaction of the
PPAR-g crystals and the PPARg–SRC1–rosiglitazone crystals, respectively.
RXR AF-2 helix with the LXXLL-binding pocket of its dimeric
Both detectors are mounted on a Rigaku RU-200 rotating anode generator.
partner. There is evidence for this allosteric mechanism and for
Data reduction, merging and scaling were done with the programs DENZO and
competition of AF-2 binding with SRC-1 (ref. 37).
Together with the structures of the apo-PPAR-g LBD and of the
Phase determination and structure refinement. For the PPAR-g multiple
ternary complex, these results indicate that ligand-dependent acti-
isomorphous replacement (MIR) structure determination, single isomorphous
vation may involve displacement of the AF-2 helix of a dimeric
replacement (SIR) phases from the phenyl mercury acetate (PMA) heavy-atom
partner from the LXXLL-binding pocket. Because the AF-2 domains
data were used to calculate a difference map of the selenomethionyl-PPAR-g
of many nuclear receptors are strikingly similar, in both length and
data. We identified 12 selenium sites in total, with 6 sites for each individual
sequence, to LXXLL motifs, this type of interaction may be relevant
monomer of the PPAR-g homodimer. With this information, the non-
to the mechanisms of ligand-dependent activation for many of these
crystallographic two-fold axis was uniquely determined and used in
proteins (Table 2).
combination with the MIR phases for electron-density averaging. MIR
There are several subtle differences between the configurations of
phases were calculated using PHASES44 and improved by solvent flattening
the ‘active' AF-2 domain in the apo-PPAR-g LBD structure and the
and electron-density averaging in program DM45,46. We built the apo-PPAR-g
AF-2 domains of the ternary complex that may explain why the
model using the program Quanta (Molecular Simulations) and refined the
binding of ligand would result in a relative preference for binding
model in X-PLOR47.
the LXXLL co-activator motif. Thiazolidinedione binding generates
The PPARg–SRC1–rosiglitazone ternary complex structure was solved by
two key hydrogen bonds between H323 and H449 that essentially
conventional molecular replacement methods using the unliganded PPAR-g
lock the AF-2 domain into the charged-clamp configuration and
structure, with X-PLOR. Molecular-replacement phases were calculated in the
alter the sidechain positions in the AF-2 domain that forms the
package CCP4 (ref. 46) using SFALL and SIGMAA, and were improved before
LXXLL-binding pocket. Because the differences between the con-
model building by solvent flattening and density averaging across the PPAR-g
formations of the apo ‘active' AF-2 domain and the ternary complex
dimer axis with the program DM. Once the SRC1-binding site was determined,
AF-2 domain are quite small, it is likely that such changes could be
residues within 5 A˚ of the site were excluded from the averaging masks. The
achieved by structurally distinct ligands, indicating a potential basis
resulting electron density was used to identify and build the structure of the
for the mechanism of action of nuclear-receptor antagonists and
SRC-1 protein, rosiglitazone and residues of PPAR-g around the LXXLL-
partial agonists.
binding pocket. The unique density for each HD1 and HD2 motif relative to the
These results suggest that LXXLL motifs are vital in mediating
PPAR homodimer is due to three crystal packing interactions involving SRC-1.
cooperative assembly of co-activator complexes on homodimeric
We have obtained other holo complexes of thiazolidinediones with PPAR-g
and heterodimeric nuclear receptors.
without SRC-1 at a lower resolution than the ternary complex presented, and
Nature Macmillan Publishers Ltd 1998
NATURE VOL 395 10 SEPTEMBER 1998
they are not significantly different from the ternary structure. The SRC-1 model
26. Voegel, J. J., Heine, M. J. S., Zechel, C., Chambon, P. & Gronemeyer, H. TIF2, a 160 kDa transcriptional
mediator for the ligand-dependent activation function AF-2 of nuclear receptors. EMBO J. 15, 3667–
was built using the software package O (ref. 48), and refined using X-PLOR47.
3675 (1996).
27. Torchia, J. et al. The transcriptional co-activator p/CIP binds CBP and mediates nuclear-receptor
Received 8 May; accepted 29 July 1998.
function. Nature 387, 677–684 (1997).
1. Mangelsdorf, D. J. et al. The nuclear receptor superfamily: the second decade. Cell 83, 835–839 (1995).
28. Zhu, Y., Qi, C., Calandra, C., Rao, M. S. & Reddy, J. K. Cloning and identification of mouse steroid
2. Kliewer, S. A. et al. Fatty acids and eicosanoids regulate gene expression through direct interactions
receptor coactivator-1 (mSRC-1), as a coactivator of peroxisome proliferator-activated receptor g.
with peroxisome proliferator-activated receptors a and g. Proc. Natl Acad. Sci. USA 94, 4318–4323
Gene Expr. 6, 185–195 (1996).
29. Hong, H., Kohli, K., Garabedian, M. J. & Stallcup, M. R. GRIP1, a transcriptional coactivator for the
3. Forman, B. M., Chen, J. & Evans, R. M. Hypolipidemic drugs, polyunsaturated fatty acids, and
AF-2 transactivation domain of steroid, thyroid, retinoid, and vitamin D receptors. Mol. Cell. Biol. 17,
eicosanoids are ligands for peroxisome proliferator-activated receptors a and d. Proc. Natl Acad. Sci.
2735–2744 (1997).
USA 94, 4312–4317 (1997).
30. Anzick, S. L. et al. AIB1, a steroid receptor coactivator amplified in breast and ovarian cancer. Science
4. Kliewer, S. A. et al. Differential expression and activation of a family of murine peroxisome
277, 965–968 (1997).
proliferator-activated receptors. Proc. Natl Acad. Sci. USA 91, 7355–7359 (1994).
31. Chen, H. et al. Nuclear receptor coactivator ACTR is a novel histone acetyltransferase and forms a
5. Chawla, A., Schwartz, E. J., Dimaculangan, D. D. & Lazar, M. A. Peroxisome proliferator-activated
multimeric activation complex with P/CAF and CBP/p300. Cell 90, 569–580 (1997).
receptor g: adipose-predominant expression and induction early in adipocyte differentiation.
32. Kamei, Y. et al. A CBP integrator complex mediates transcriptional activation and AP-1 inhibition by
Endocrinol. 135, 798-800 (1994).
nuclear receptors. Cell 85, 403–414 (1996).
6. Willson, T. M. et al. The structure-activity relationship between peroxisome proliferator-activated
33. Voegel, J. J. et al. The coactivator TIF2 contains three nuclear receptor-binding motifs and mediates
receptor g agonism and the antihyperglycemic activity of thiazolidinediones. J. Med. Chem. 39, 665–
transactivation through CBP binding-dependent and -independent pathways. EMBO J. 17, 507–519
7. Wu, Z., Bucher, N. L. R. & Farmer, S. R. Induction of peroxisome proliferator-activated receptor g
34. Heery, D. M., Kalkhoven, E., Hoare, S. & Parker, M. G. A signature motif in transcriptional co-
during the conversion of 3T3 fibroblasts into adipocytes is mediated by C/EBPb, C/EBPd, and
activators mediates binding to nuclear receptors. Nature 387, 733–736 (1997).
glucocorticoids. Mol. Cell. Biol. 16, 4128–4136 (1996).
35. Keen, H. Insulin resistance and the prevention of diabetes mellitus. New. Eng. J. Med. 331, 1226–1227
8. Tontonoz, P., Hu, E., Graves, R., Budavari, A. & Spiegelman, B. mPPARg2: tissue-specific regulator of
an adipocyte enhancer. Genes Dev. 8, 1224–1234 (1994).
36. Buckle, D. R. et al. Non-thazolidinedione antihyperglycemic agents. 2: a-Carbon substituted b-
9. Tontonoz, P., Hu, E. & Spiegelman, B. M. Stimulation of adipogenesis in fibroblasts by PPARg2, a lipid
phenylpropanoic acids. Bioorg. Med. Chem. Lett. 6, 2127–2130 (1996).
activated transcription factor. Cell 79, 1147–1156 (1994).
37. Westin, S. et al. Interactions controlling complexes of nuclear-receptor heterodimers and co-
10. Ricote, M., Li, A. C., Willson, T. M., Kelly, C. J. & Glass, C. K. The peroxisome proliferator-activated
activators. Nature 395, 199–202 (1998).
receptor g is a negative regulator of macrophage activation. Nature 391, 79–82 (1998).
38. Feng, X. et al. Suprabasal expression of a dominant-negative RXR alpha mutant in transgenic mouse
11. Tontonoz, P., Nagy, L., Alvarez, J. G. A., Thomazy, V. A. & Evans, R. M. PPARg promotes monocyte/
epidermis impairs regulation of gene transcription and basal keratinocyte proliferation by RAR-
macrophage differentiation and uptake of oxidized LDL. Cell 93, 241–252 (1998).
selective retinoids. Genes Dev. 11, 59–71 (1997).
12. Brun, R. P. et al. Differential activation of adipogenesis by multiple PPAR isoforms. Genes Dev. 10,
39. Durand, B. et al. Activation function 2 (AF-2) of retinoic acid receptor and 9-cis retinoic acid receptor:
974–984 (1996).
presence of a conserved autonomous constitutive activating domain and influence of the nature of the
13. Lehmann, J. M. et al. An antidiabetic thiazolidinedione is a high affinity ligand for peroxisome
response element on AF-2 activity. EMBO J. 13, 5370–5382 (1994).
proliferator-activated receptor g (PPARg). J. Biol. Chem. 270, 12953–12956 (1995).
40. Henttu, P. M. A., Kalkhoven, E. & Parker, M. G. AF-2 activity and recruitment of steroid receptor
14. Hulin, B., McCarthy, P. A. & Gibbs, E. M. The glitazone family of antidiabetic agents. Curr. Pharm.
coactivator 1 to the estrogen receptor depend on a lysine residue conserved in nuclear receptors. Mol.
Des. 2, 85–102 (1996).
Cell. Biol. 17, 1832–1839 (1997).
15. Evans, R. M. The steroid and thyroid hormone receptor superfamily. Science 240, 889–895 (1988).
41. Kurokawa, R. et al. Regulation of retinoid signalling by receptor polarity and allosteric control of
16. Bourguet, W., Ruff, M., Chambon, P., Gronemeyer, H. & Moras, D. Crystal structure of the ligand-
ligand binding. Nature 371, 528–531 (1994).
binding domain of the human nuclear receptor RXR-a. Nature 375, 377–382 (1995).
42. Forman, B. M., Umesono, K., Chen, J. & Evans, R. M. Unique response pathways are established by
17. Wagner, R. L. et al. A structural role for hormone in the thyroid hormone receptor. Nature 378, 690–
allosteric interactions among nuclear hormone receptors. Cell 81, 541–550 (1995).
43. Otwinowski, Z., Isaacs, N. & Burley, S. in Proc. CCP4 Study Weekend (ed. Sawyer, L.) 56–62 (SERC
18. Renaud, J.-P. et al. Crystal structure of the RAR-g ligand-binding domain bound to all-trans retinoic
Caresbury Lab., Daresbury, 1993).
acid. Nature 378, 681–689 (1995).
44. Furey, W. S. & Swaminathin, S. in Methods in Enzymology: Macromolecular Crystallography Part B Vol.
19. Brzozowski, A. M. et al. Molecular basis of agonism and antagonism in the oestrogen receptor. Nature
277 (eds Carter, C. & Sweet, R.) 590–620 (Academic, Orlando, 1997).
389, 753–758 (1997).
45. Cowtan, K. ‘‘DM'': an automated procedure for phase improvement by density modification. Joint
20. Horwitz, K. B. et al. Nuclear receptor coactivators and corepressors. Mol. Endocrinol. 10, 1167–1177
CCP4 ESF-EACBM Newsletter Protein Crystallogr. 31, 34–38 (1994).
46. Collaborative Computational Project Number 4. The CCP4 suite: programs for protein
21. Glass, C. K., Rose, D. W. & Rosenfeld, M. G. Nuclear receptor coactivators. Curr. Opin. Cell Biol. 9,
crystallography. Acta Cryst. D 50, 760–776 (1994).
222–232 (1997).
47. Brunger, A. X-PLOR Version 3.0: A System for Crystallography and NMR (Yale Univ. Press, New Haven,
22. Barettino, D., Vivanco Ruiz, M. M. & Stunnenberg, H. G. Characterization of the ligand-dependent
transactivation domain of thyroid hormone receptor. EMBO J. 13, 3039–3049 (1994).
48. Jones, T. A., Zou, J. Y., Cowan, S. W. & Kjeldgaard, M. Improved methods for building protein models
23. Danielian, P. S., White, R., Lees, J. A. & Parker, M. G. Identification of a conserved region required for
in electron density maps and the location of errors in these models. Acta Cryst. A 47, 110–119 (1991).
hormone-dependent transcriptional activation by steroid hormone receptors. EMBO J. 11, 1025–
1033 (1992).
Acknowledgements. We thank W. Burkhart, M. Moyer and K. Blackburn for protein sequencing and
mass spectrometry; S. Blanchard, P. Charifson, T. Consler, S. Jordan, S. Kliewer, J. Lehmann, C. Mohr and
24. Durand, B. et al. Activation function 2 (AF-2) of retinoic acid receptor and 9-cis retinoic acid receptor;
E. Xu for discussions; A. Miller for artwork; and K. Milburn for computational assistance.
presence of a conserved autonomous constitutive activating domain and influence of the nature of the
response element on AF-2 activity. EMBO J. 13, 5370–5382 (1994).
Correspondence and requests for materials should be addressed to M.V.M. (e-mail: mvm25452@
25. Tone, Y., Collingwood, T. N., Adams, M. & Chatterjee, V. K. Functional analysis of a transactivation
glaxowellcome.com). Coordinates for the apo-PPAR-g (1PRG) and the PPARg–rosiglitazone–SRC1
domain in the thyroid hormone beta receptor. J. Biol. Chem. 269, 31157–31161 (1994).
ternary complex (2PRG) have been deposited with the Brookhaven Protein Database.
Nature Macmillan Publishers Ltd 1998
NATURE VOL 395 10 SEPTEMBER 1998
Source: http://space.geocities.jp/idensikouzou/book/Nature-article.395.137-143.1998.pdf
Sommaire 1) Ce dont traite ce document 2) Avant le départ 3) Les vaccins 4) La pharmacie du bord, un casse-tête à ranger 5) Règles simples pour éviter que les menus bobos deviennent de gros problèmes. Repousser les microbes Partir en balade Chasser le hoquet Chasser le rhume Se prémunir contre les chocs Aiguilles d'oursins Piqûres d'abeilles
PREVENTION DES COMPLICATIONS DE LA CORTICOTHERAPIE GENERALE DANS LES PEMPHIGUS DE L'ADULTE Catherine Prost Squarcioni Centre de Références Maladies Rares NET-DBAI-IDF Hôpital Saint Louis et hôpital Avicenne La cortisone, découverte en 1935, a transformé l'évolution de nombreuses maladies inflammatoires et auto-immunes (en particulier celle des pemphigus) qui étaient auparavant constamment mortelles. Depuis, la cortisone a été abandonnée au profit de nouvelles molécules encore plus efficaces et provoquant moins d'effets secondaires ; de nombreux progrès ont été faits pour prévenir ces derniers. Avant d'aborder précisément ce sujet, quelques remarques pour expliquer notre jargon médical et notre démarche thérapeutique dans les pemphigus. La corticothérapie générale s'utilisant de la même manière dans les différentes formes de pemphigus, en particulier dans les pemphigus vulgaires et les pemphigus superficiels, nous parlerons de pemphigus sans plus de précision. • On entend par corticothérapie générale (ou systémique) l'administration de corticoïdes par voie orale (par la bouche), intramusculaire ou intraveineuse (piqûres). En France, nous utilisons indifféremment :