Doi:10.1016/j.neuron.2008.03.016
Role of Olivary Electrical Couplingin Cerebellar Motor Learning
Ruben S. Van Der Giessen,Sebastiaan K. Koekkoek,Stijn van Jornt R. De Gruijl,Alexander Cupido,Sara Bjorn Kerstin Wellershaus,Joachim Degen,Jim Deuchars,Elke C. Fuchs,Hannah Monyer,Klaus Marcel T.G. De and Chris I. De ,1Department of Neuroscience, Erasmus MC, 3000 DR Rotterdam, The Netherlands2Institute of Genetics, Division of Molecular Genetics, University of Bonn, 53117 Bonn, Germany3Institute of Membrane and Systems Biology, University of Leeds, LS2 9JT Leeds, UK4Department of Clinical Neurobiology, Interdisciplinary Center for Neuroscience, 69120 Heidelberg, Germany5Netherlands Institute for Neuroscience, Royal Academy of Arts and Sciences (KNAW), 1105 BA Amsterdam, The Netherlands6These authors contributed equally to this work.
*Correspondence: DOI 10.1016/j.neuron.2008.03.016
distribution of Cx36 and/or to create mouse mutants to investi-gate the cellular consequences of a lack of Cx36 in the brain
The level of electrotonic coupling in the inferior olive
is extremely high, but its functional role in cerebellar
Cx36 and neuronal gap junctions are widely distributed in re-
motor control remains elusive. Here, we subjected
gions such as the olfactory bulb, hippocampus, cerebral cortex,
mice that lack olivary coupling to paradigms that
(hypo)thalamus, and inferior olive ). For
require learning-dependent timing. Cx36-deficient
most of these regions, the possible role of neuronal gap junc-
mice showed impaired timing of both locomotion
tions has been determined at the cell-physiological level (); In these
and eye-blink responses that were conditioned to
in vitro studies, a lack of Cx36 generally leads to an absence of
a tone. The latencies of their olivary spike activities
electrotonic coupling and to changes in subthreshold activities
in response to the unconditioned stimulus were sig-
nificantly more variable than those in wild-types.
for most of the brain systems mentioned above, the apparent
Whole-cell recordings of olivary neurons in vivo
behavioral phenotype is relatively mild and/or remains a topic
showed that these differences in spike timing result
for systems electrophysiological investigations
at least in part from altered interactions with their
subthreshold oscillations. These results, combined
, for studies on retina). With regard to the
with analyses of olivary activities in computer simula-
olivocerebellar system, previous behavioral studies on
Cx36/
tions at both the cellular and systems level, suggest
mutants showed no ataxia and a relatively normal motor perfor-
that electrotonic coupling among olivary neurons
mance (This lack of a clear phenotype dur-ing natural motor behavior is remarkable, because in mammals
by gap junctions is essential for proper timing of their
the density of gap junctions in the inferior olive is probably
action potentials and thereby for learning-dependent
higher than in any other brain region
timing in cerebellar motor control.
Here, we show that, although
Cx36/ mutants have no prom-inent general motor deficits, they do show problems when
challenged to perform a learning-dependent motor task suchas conditioning their locomotion pattern or eye-blink response
More than a century ago, in 1906, Santiago Ra´mon y Cajal
to a tone. In these learning tasks, the timing of the motor re-
received the Nobel Prize for the neuron doctrine stating that
sponses is modified by conditioning the movement to a condi-
neurons operate as anatomically and functionally distinct cellu-
tioned stimulus (CS) that starts before, but coterminates with,
lar units in the mammalian brain. This tenet still holds, but over
an unconditioned stimulus (US) (
the past decade the neuron doctrine has been complemented
The CS is probably
by new discoveries about the constitution, distribution, and
conveyed by the mossy fiber-parallel fiber system to the Pur-
cell-physiological functions of neuronal gap junctions that can
kinje cells in the cerebellar cortex, while the US is conveyed
establish cytoplasmic continuity among large ensembles of
by their climbing fibers originating from the inferior olive (
neurons Importantly, in 1998, groups led
). Thus, in the current
by Condorelli (and Willecke (
study we investigated the hypothesis that appropriate timing of
cloned the first gap junction protein, i.e., connexin36
conditioned motor responses critically depends on the precise
(Cx36), that is predominantly expressed by neurons. The iden-
temporal coding of the activities of coupled neurons in the
tification of this protein allowed several groups to study the
inferior olive.
Neuron
58, 599–612, May 22, 2008 ª2008 Elsevier Inc. 599
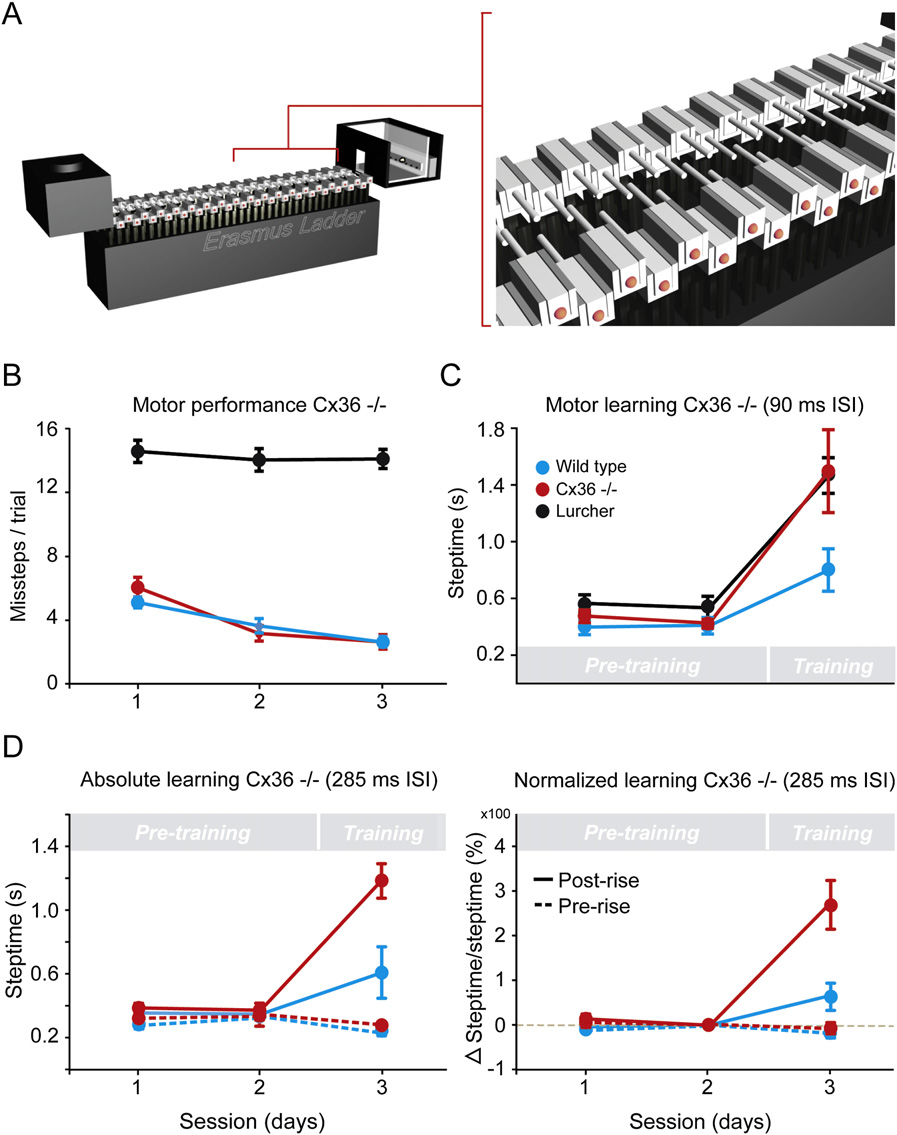
Role of Olivary Coupling in Learning
Figure 1. Erasmus Ladder Test for Detect-ing Performance and Learning Deficits inLocomotion(A) The ladder consists of 2 3 37 horizontal rungsplaced in between two shelter boxes. Mice aretrained to walk from one side to the other usingair pressure devices placed in the bottom of theboxes. The rungs, which are all equipped withpressure sensors, can be moved up or downunder the control of a linked computer systemthat can analyze the walking pattern instanta-neously during locomotion.
(B) Motor performance level is revealed by theamount of descended rungs touched, which rep-resents the number of missteps. Cx36/ mutants(red) and wild-type littermates (blue) show normalmotor performance, while lurcher mice (black)show deficits in performance during all sessions.
(C) Motor learning level is revealed by the changein steptime after and before conditioning (i.e.,training versus pretraining). During conditioningtrials, a randomly selected rung rises 12 mm abovethe walking level to create a perturbation; thisunconditioned stimulus occurs at a fixed moment(interstimulus interval [ISI]) after the onset of theconditioned stimulus (a 15 kHz tone). BothCx36/ mutants and lurcher mice reveal difficul-ties in motor learning.
(D) While the data obtained in (C) have beenobtained with an ISI of 90 ms, these data havebeen obtained with an ISI of 285 ms. Note thatthe deficits in learning-dependent timing are justas pronounced with this longer ISI, regardlesswhether they are revealed in an absolute (left) ornormalized (right) fashion. The normalized datashow values with respect to those obtained in ses-sion 1. Also note that the steptimes in the phasepreceding the rise of the rung (prerise) are not pro-longed during the training (dotted curves). Valuesindicate mean ± SD.
In this paradigm, the Cx36/ mutants and wild-type littermatesshowed a similar overall average steptime, which was defined as
Deficits in Locomotion Conditioning
the time needed to place the front paw from one rung to the other
To quantify their general level of motor performance, Cx36/
(358 ms ± 29 SD for Cx36/ mutants and 339 ms ± 23.5 SD for
mutants (C57BL/6 background, n = 16) and wild-type littermates
wild-types), and a comparably low number of missteps, which
(n = 18) were trained to walk on the Erasmus ladder. The Eras-
were identified by touches on the descended rungs (p > 0.10
mus ladder is formed by 2 3 37 rungs positioned between a start
for each session, t test; For comparison, we also
and end box across which the mice can run back and forth
tested lurcher mice (C57BL/6 background, n = 9), which lack
(left panel) (see section A available
Purkinje cells and are known to show cerebellar ataxia
online). Each rung on both the left and right side is equipped with
a pressure sensor, which is continuously monitored. Based on
spontaneous mouse mutants showed longer steptimes (589 ms
instantaneous analysis of the activities of these sensors, the
± 49 SD; p < 0.01 in both cases, t tests) and three to four times
walking pattern of the mice can be predicted in the millisecond
more missteps than Cx36/ mutants or controls (p < 0.0001
range and, if wanted, interrupted by moving each individual
and p < 0.0001 for each session, t tests). Thus, in line with previ-
rung up or down. Initially, the mice were trained with the even-
ous rotarod tests (), we con-
numbered rungs on the left side and the odd-numbered rungs
clude from this test on the Erasmus ladder that Cx36/ mutants
on the right side in a descended position so as to create an alter-
show, in contrast to lurcher mice, a relatively normal motor
nated stepping pattern with 30 mm gaps right panel).
600 Neuron 58, 599–612, May 22, 2008 ª2008 Elsevier Inc.
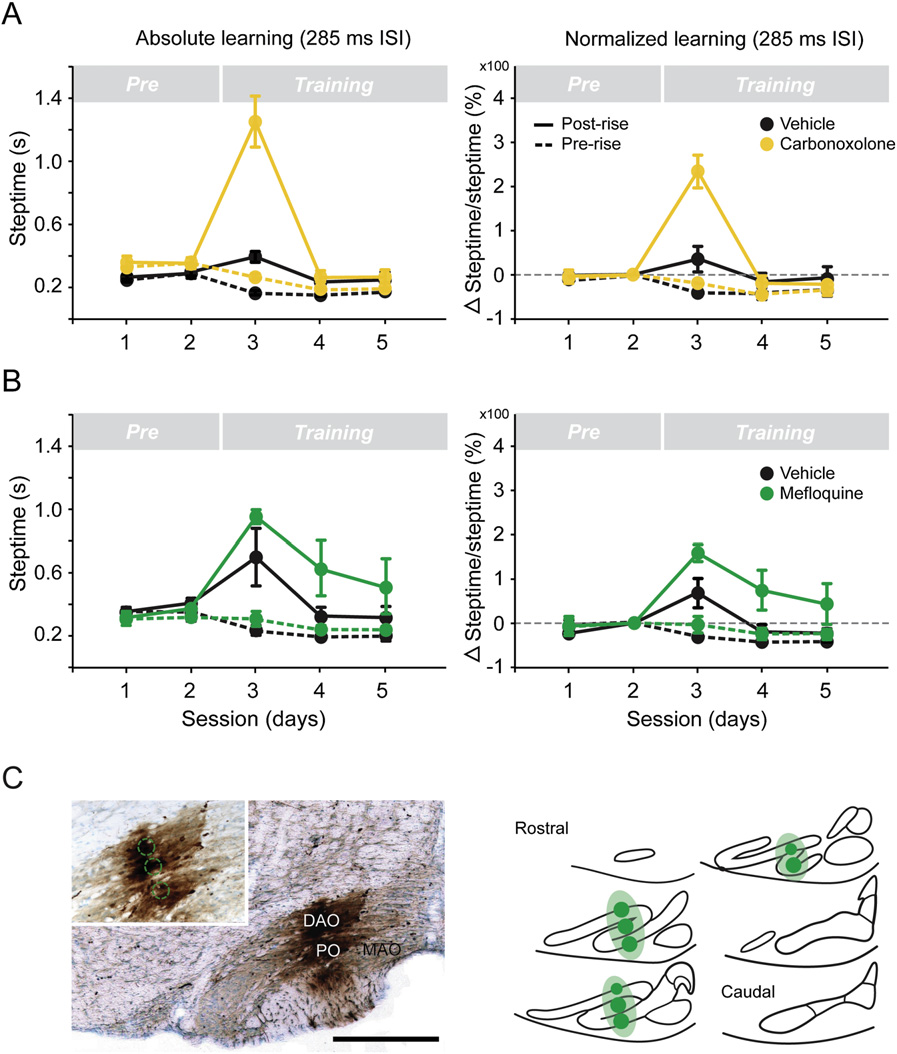
Role of Olivary Coupling in Learning
Figure 2. Systemic Applications of Carbe-noxolone or Intraolivary Injections of Meflo-quine Affect Learning-Dependent Timing(A) Carbenoxolone was injected i.p. just before thetwo motor performance sessions (Pre) and justbefore the first of a series of three conditioningsessions (Training).
(B) Mefloquine was injected bilaterally inside theinferior olive 1 day before the series of the threeconditioning sessions.
(C) Example of one of the reconstructions of theinjection site in the olive following BDA labeling.
Values indicate mean ± SD.
for side effects seeWe either applied carbenoxolonesystemically (40 mg/kg) just before twomotor performance sessions and justbefore the first of a series of three condi-tioning sessions (n = 5), or we made in-traolivary
(150 mM) 1 day before a series of threeconditioning sessions (n = 4) In these experiments, we alsofound a significantly greater increase inthe front paw steptime of the coupling-deficient animals after, but not before,conditioning than in control animals (n =6 and 5, respectively), which received in-jections with vehicle only (p < 0.002 andp < 0.001, respectively, t tests) In the case of carbenoxolone,the impact of the drug on conditioningtapered off more quickly than that ob-
To find out whether the ability for motor learning is affected in
served after the mefloquine injections (at session four, p <
Cx36/ mice, we subjected them to a conditioning paradigm in
0.05, t test); this difference may be due to a relatively strong
which they were trained to make a new locomotion movement
and fast clearance of carbenoxolone
using a 15 kHz tone as the CS and a rising rung as the US (ISI
of 90 ms; see section A). A training session
Taken together, the experiments on the lurcher mice and on
consisted of eight blocks of eight trials, which were separated
the animals subjected to intraolivary injections described above
by an intertrial interval of 8–12 s. As described above for the initial
showed that the Erasmus ladder allows us to detect specific
motor performance test, during the preconditioning sessions,
deficits in both cerebellar motor performance (lurcher) and cere-
the steptime was not different among mutants and controls
bellar motor learning (pharmaceutical manipulation of the olive).
(p > 0.25, t test). However, as soon as the conditioning procedure
We can therefore conclude that genetic or pharmaceutical
started, the front paw steptime in the mutants increased signifi-
blocking of olivary coupling mediated by Cx36 has relatively little
cantly compared to that of controls (p < 0.05, t test). In fact, this
effect on cerebellar motor performance, while it probably does
increase in steptime was comparable to that of lurchers
affect cerebellar motor learning in that the training process is
(C). The difference among mutants and wild-types re-
slowed down substantially.
mained when we prolonged the interstimulus (CS – US) intervals(ISIs) from 90 ms to 285 ms (p < 0.001, t test) D).
Deficits in Eye-Blink Conditioning
Because the Cx36/ mutants lack Cx36 from early on and
Analysis of the locomotion conditioning process described
may therefore show compensations within the olivary neurons
above suggests that learning-dependent timing is affected in
(we also tested the same conditioning
Cx36/ mice. However, in this paradigm, the movements are an-
Erasmus ladder paradigm in wild-type mice following application
alyzed in discrete steps, which makes it virtually impossible to
of drugs that can block olivary coupling instantaneously (
identify the exact deficits over time during continuously
recorded ongoing locomotion movements. Thus, to further
Neuron 58, 599–612, May 22, 2008 ª2008 Elsevier Inc. 601
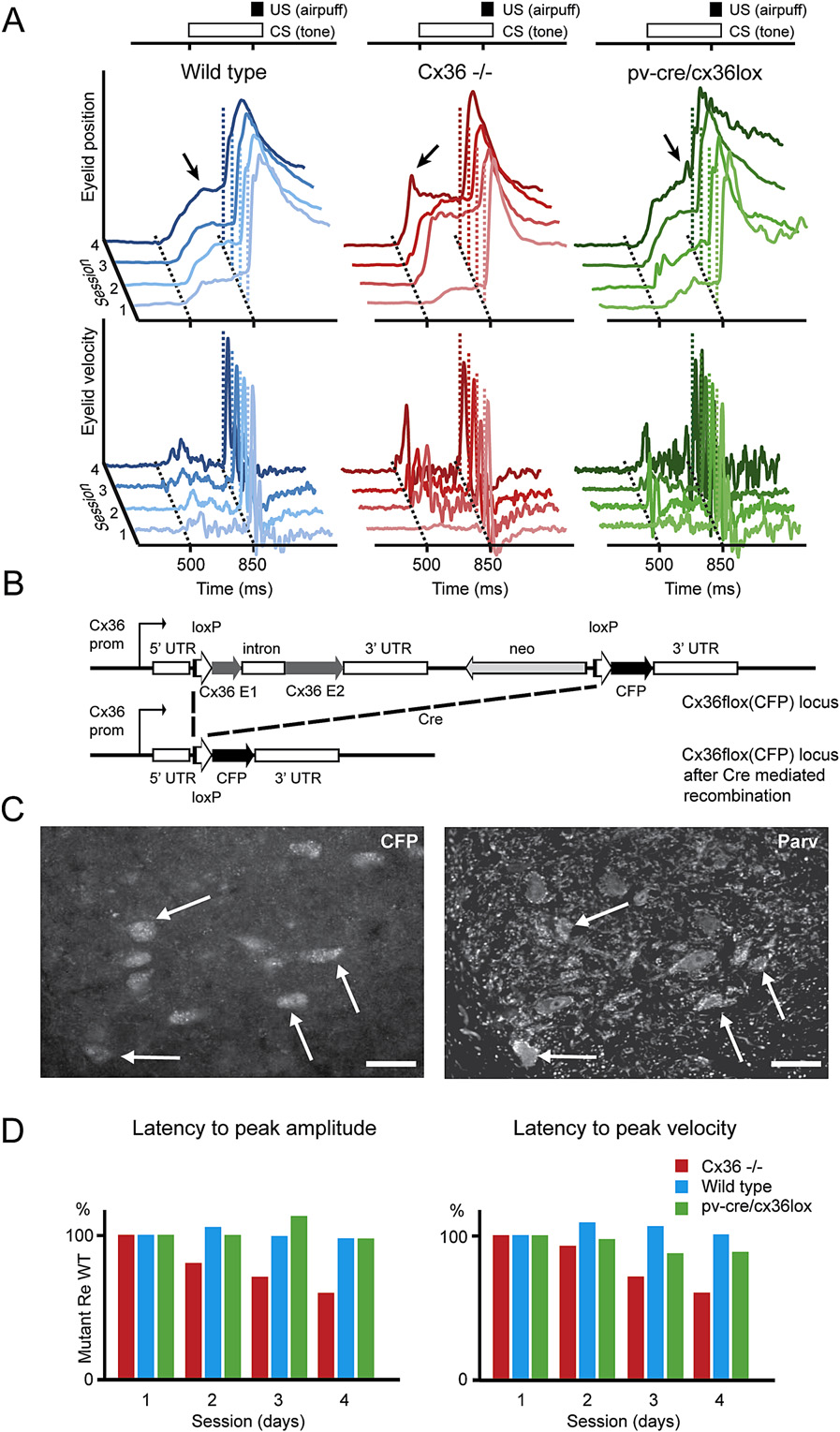
Role of Olivary Coupling in Learning
Figure 3. A Lack of Coupling by Cx36 GapJunction Proteins in the Inferior Olive, butNot in the Cerebellar Nuclei, Results inImpaired Learning-Dependent Timing(A) Representative examples of eye-blink traces ina wild-type (blue), global Cx36/ mutant (red),and Cx36 del(lacZ)/flox(CFP): parvalbumin-Crecontrol mouse (green). They all show conditionedeye-blink responses after four training sessionsusing a tone as the conditioned stimulus (CS)and an air puff as the unconditioned stimulus(US). However, while the timing of the learnedresponse in wild-type and floxed-Cre controlmice improves over the sessions, that in the globalCx36/ mutants gets worse (see arrows).
(B) Genetic design of floxed-Cx36 mutants cross-bred with parvalbumin-Cre mice, which were usedas controls.
(C) The Cx36 del(lacZ)/flox(CFP): parvalbumin-Crecontrol mice showed CFP staining in their cerebel-lar nuclei neurons, demonstrating that Cx36 hasbeen floxed in these neurons and thus not ex-pressed. Immunostaining for parvalbumin of thesame sections (right panel) showed that most ofthe cerebellar nuclei neurons indeed express par-valbumin (arrows) and that they would otherwise inthe nonfloxed situation express Cx36. Thus, theCx36 del(lacZ)/flox(CFP): parvalbumin-Cre controlmice do not express Cx36 in their cerebellar nu-clei, while their expression in the relevant olivarysubnuclei is normal (data not shown). Punctatelabeling in neuropil of the right panel reflects stain-ing of axonal fibers.
(D) While the latency to peak amplitude (left panel)in the global knockouts of Cx36 (red) got worseduring the training sessions, that in the Cx36del(lacZ)/flox(CFP): parvalbumin-Cre control mice(green) was indistinguishable from that in theirunaffected littermates (blue). Similarly, the averagelatency to peak velocity (right panel) in the mutantswas also significantly reduced (p < 0.01; t test).
wild-type littermates (n = 8) to a condition-ing paradigm using a tone as the CS andan air puff as the US at ISIs of 350 ms.
Here, too, the eight daily training blocksconsisted of eight trials (one US alone,six paired, and one CS alone). After fourpaired training sessions (T-1 to T-4), thepercentage of conditioned responsesand their average peak amplitude inwild-types reached levels of 77% and0.48 mm, respectively, while those inCx36/ mice had values of 78% and0.56 mm. These values were not signifi-cantly different (p > 0.9 and p > 0.5;
investigate the potential role of Cx36 in learning-dependent
MANOVA). In contrast, the timing properties of the conditioned
timing, we compared the Cx36/ mutants with wild-type litter-
responses differed dramatically among wild-types and mutants
mates in an eye-blink conditioning paradigm. For this paradigm,
as the training proceeded. While in wild-types the average
the motor responses can be continuously measured using the
latency to peak amplitude of the conditioned responses was
magnetic distance measurement technique (MDMT;
appropriately fixed at the moment when the US occurs, the timing
We subjected adult Cx36/ mutants (n = 8) and
in mutants got worse during the four training sessions
602 Neuron 58, 599–612, May 22, 2008 ª2008 Elsevier Inc.
Role of Olivary Coupling in Learning
and section B). At the end of the training, the
lobe In awake wild-types (n = 9), the air puff stimula-
average latency to peak amplitude in the mutants preceded the
tion evoked virtually only short-latency climbing fiber responses;
moment of the US by 196 ms (±27 SD), which was significantly
these responses had an average peak latency of 29 ± 9 ms (SD,
different from that in controls (80 ms ± 24 SD; p < 0.005, t test)
averaged over the nine animals) over 879 successful stimulations
(see also D). These data indicate that coupling-deficient
(>70% successful). In contrast, in all Purkinje cells recorded in
mutants cannot appropriately time their movements when chal-
the Cx36/ mutants (n = 10), the same perioribital stimulation
lenged in a conditional task.
evoked both short-latency responses (average to peak of 30 ±
Still, these eye-blink experiments on the global Cx36 null
7 ms) and long-latency responses (101 ± 17 ms) B). Of
mutants do not directly demonstrate that the behavioral pheno-
all successful stimulations (n = 1036, >75% successful) in the
type can be attributed solely to a lack of coupling in the inferior
mutants, 60% and 34% resulted in pure short-latency and
olive. GABAergic neurons in many brain regions, including those
pure long-latency responses, respectively, while 6% resulted
in the cerebellar nuclei, also show a prominent expression of
in both a short-latency and long-latency response. These differ-
ences in the temporal distribution patterns following peripheral
a lack of this expression may therefore in principle also affect
stimulation were highly significantly different among wild-types
cerebellar conditioning. To address this potential caveat, we
and mutants (p < 0.001, t tests). Similarly, during spontaneous
generated mutant mice in which the expression of Cx36 is
activity in the awake state, the Cx36/ mutants showed signif-
ablated in the GABAergic neurons of the brain, while that in the
icantly (p < 0.01, t test) more doublets of two or three climbing
relevant olivary neurons, which are all non-GABAergic, is normal
fiber responses occurring within 200 ms (12% ± 6%, SD) than
(and 3C). These mutants (n = 4), which were cross-
wild-types (5% ± 2%, SD) (C, upper panel), while their
breedings of floxed-Cx36 mutants and parvalbumin-Cre mice,
mean interspike interval within a doublet was significantly
lacked expression of Cx36 in, for example, the GABAergic neu-
smaller (123 ± 11 ms in Cx36/ mutants versus 140 ± 12 ms
rons of the thalamus, olfactory bulb, cerebral cortex, hippocam-
in wild-types; p < 0.01, t test) middle panel). This dif-
pus, and cerebellar nuclei that express parvalbumin
ference was also reflected by a generally increased coefficient of
whereas the neurons in the dorsal
variation for spike intervals (p < 0.02, t test) (C, bottom
accessory olive that are involved in eye-blink conditioning
panel). All these differences in complex spike activities were
showed a normal level of Cx36. The pv-cre/cx36lox cross-bred
not influenced by differences in average firing frequencies, be-
mutants showed the same conditioned responses as wild-types,
cause the average complex spike frequency was not signifi-
i.e., without any deficits in their timing (A and 3D). These
cantly different among wild-types and Cx36/ mutants (0.94 ±
data indicate that in the global Cx36/ knockout it is most likely
0.19 versus 1.00 ± 0.26; p > 0.4, t test; cf.
the deficits in the olive that are responsible for the abnormalities
, for differences in anesthetized state). Likewise, the shape
in learning-dependent timing. For the same argument, it is also
of the climbing fiber responses and the average number of spike-
relevant to find out whether coupling among stellate cells in the
lets within the complex spikes (B) as well as the average
cerebellar cortex is affected in the Cx36 mutant mice
firing rate (67 ± 13 versus 69 ± 14) and coefficient of variation
). We therefore investigated the consti-
(0.68 ± 0.2 versus 0.57 ± 0.2) of simple spike activities of the mu-
tution of the gap junctions between these cells. It turned out that
tants were also not different from those in wild-types. Thus, Pur-
the vast majority of these interneuronal gap junctions are formed
kinje cells in awake mice lacking Cx36 show robust differences in
by connexin45 (see in
the temporal pattern of their climbing fiber responses, but not in
rather than Cx36 (Thus, we con-
the average firing frequencies of their ongoing activities.
clude that Cx36/ mutants show deficits in learning-dependenttiming and that this behavioral deficit is likely due to an impair-
Altered Correlation between Spiking Activities
ment of electrotonic coupling in the inferior olive.
and Subthreshold OscillationsTo explain the differences in latencies and spiking patterns, we
Abnormal Temporal Pattern of Climbing
investigated the activities of olivary neurons using whole-cell
recordings in vivo in anesthetized animals (). The majority
If the deficits in learning-dependent timing are due to a lack of
of the neurons in wild-types showed pronounced subthreshold
coupling between the olivary neurons, one would expect that
oscillations that either had a clear sinusoidal appearance,
the timing properties of the activities in these neurons would
a more complex rhythmic shape, which probably corresponds
be disrupted in Cx36/ mutants. To investigate these proper-
to the activation of low-threshold calcium conductances
ties, we recorded the climbing fiber activities of Purkinje cells
), or both types of subthreshold activities
in the cerebellar cortex that reveal the olivary signals of the US
(A) (see also ). In the mutants,
during eye-blink conditioning (
the same types of oscillating cells were observed, but they
The climbing fiber activities of Purkinje cells, also called
showed significantly more cells that did not oscillate (p < 0.01,
complex spikes, generally reflect the temporal coding of olivary
c2 test), and the occurrence of their oscillations depended signif-
neurons rather precisely, because they are generated in an all-
icantly stronger on the membrane potential (p < 0.01, t test), as
or-none fashion We found that the Pur-
previously described for in vitro conditions
kinje cells that respond well to air puff stimulation are in the
) (and 5C). Power spectra of
mouse situated in an area covering both lobulus simplex in the
the oscillating subthreshold activities showed that the frequen-
hemisphere and the adjacent part of lobule VI in the posterior
cies of the oscillations occurred in both wild-types and mutants
Neuron 58, 599–612, May 22, 2008 ª2008 Elsevier Inc. 603
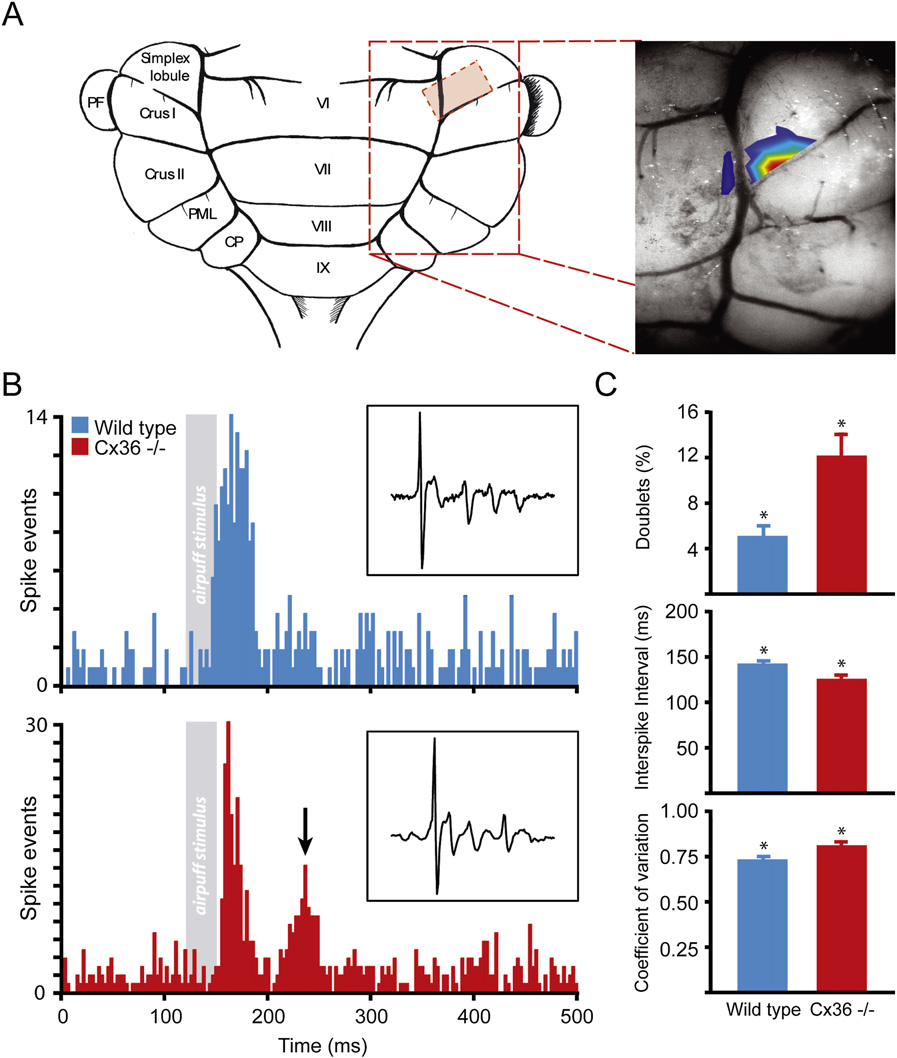
Role of Olivary Coupling in Learning
Figure 4. A Lack of Coupling of Inferior Oli-vary Neurons Results in Altered Timing ofClimbing Fiber Activities in the CerebellarCortex(A) Purkinje cells that responded well to air puffstimulation (i.e., US) were situated in an area cov-ering the lobulus simplex in the hemisphere andadjacent part of lobule VI in the posterior lobe.
Color codings correspond to percentage of Pur-kinje cells that responded with complex spikeactivities to air puff stimulation; red, yellow, green,light blue, and dark blue indicate success rates of50%, 40%, 30%, 20%, and 10%, respectively.
(B) Air puff stimulation evoked only short-latencyclimbing fiber responses in awake wild-type mice(blue) (latency of 29 ± 9 ms), while in the Cx36/mutants (red) the same perioribital stimulationevoked both short-latency (30 ± 7 ms) and long-la-tency responses (101 ± 17 ms) (arrow). Note that inboth cases units on the y axis represent two spikes.
Insets show typical complex spike responses inwild-types (top) and mutants (bottom) in 8 mstrace; no differences were observed in the shape.
(C) During spontaneous activity in the awake state,the Cx36/ mutants showed significantly moredoublets of two or three complex spikes occurringwithin 200 ms (p < 0.01, t test), a significantlysmaller mean interspike interval within these dou-blets (p < 0.01, t test), and a general increased co-efficient of variation for spike intervals (p < 0.02, ttest). Values indicate mean ± SD.
generation and subthreshold oscillationsalso occurred following peripheral stimu-lation as used in the conditioning para-digms described above (E).
Following peripheral stimulation, the fre-quency of the subsequent oscillations inthe mutant cells was significantly less sta-
mostly in the range of 1–3 Hz or in the range of 6–9 Hz (D).
ble (p < 0.02, t test) than in the wild-types (for quantification of
Similarly, action potentials of olivary cells in both wild-types and
first four cycles after stimulus see F). Thus, peripheral
mutants showed a so-called capacity to reset the subthreshold
sensory stimulation can indeed induce and modify the sub-
oscillation (see also
threshold activities, and such modulation can influence the tim-
Still, the olivary activities differed
ing of subsequent spiking activities of olivary neurons when they
in that the oscillations of the wild-type neurons often showed
are appropriately coupled. Therefore, the altered timing of the
dynamic crescendo amplitudes starting directly after the gener-
climbing fiber signals mediating the US in the coupling-deficient
ation of an action potential (crescendo amplitudes were defined
Cx36/ mutants can at least in part be explained by altered in-
as at least a 3-fold increase of the amplitude over five cycles),
teractions with their subthreshold oscillations.
while those in the mutants showed significantly more (p < 0.05, ttest) constant amplitudes A). Moreover, the relation-
How May a Lack of Coupling among Olivary Neurons
ship between the preferred frequencies of the subthreshold
in the Cx36/ Mutant Lead to Altered Spiking?
oscillations and those of the olivary spiking activities was less
If the lack of coupling between olivary neurons indeed leads to
tight in the Cx36 null mutant (mean residual scores of 1.33 versus
altered timing of their action potentials due to altered interactions
0.63; p < 0.01, t test) Interestingly, this reduced
with their subthreshold oscillations, we should be able to find
correlation is in line with the observation that the olivary spikes
similar relations and characteristics in a simulation of olivary neu-
occurred significantly more often (p < 0.05, t tests) during the
rons. To this end, we used a modified version of a two-compart-
peak period of an oscillation in wild-types than in mutants
mental olivary cell model by
(peak period is defined as the period plus or minus 90 from
and section C including videos). Because
the peak) (right panel in see also arrows in
direct responses to depolarizing pulses in the olive are generally
A). These differences in interactions between spike
not delayed for periods of time near 100 ms (
604 Neuron 58, 599–612, May 22, 2008 ª2008 Elsevier Inc.
Role of Olivary Coupling in Learning
Properties of Olivary Neurons in Wild-Type and Cx36/ Mutants following Whole-Cell Recordings In Vivo (Values Indicate
Cells Expressing Spontaneous STOs
Resting membrane potential (mV)
Input resistance (MU)
Membrane capacitance (pF)
Coefficient of variation for spike intervals
STO frequency (Hz)
STO amplitude (mV)
% Cells expressing depolarizing sag
% Cells expressing rebound depolarization
% Cells expressing afterhyperpolarization
Cells Expressing No Spontaneous STOs
Resting membrane potential (mV)
Input resistance (MU)
Membrane capacitance (pF)
Coefficient of variation for spike intervals
% Cells expressing depolarizing sag
% Cells expressing rebound depolarization
% Cells expressing afterhyperpolarization
Statistics: t test or c2 test. *All measured subthreshold oscillations (STOs); a single cell can have multiple STO frequencies.
), while long-latency responses due
Cx36/ network depended on increased excitability to be
to reverberating loops can be readily found when the network is
able to respond with at least some level of synchrony, reacting
as quickly as possible as pulses to different cells arrive in pre-
secondary response found in Cx36-deficient mice is probably
sumably rapid succession B, right panel). Simulations
caused by another input. Therefore, we applied to both the
of Cx36-deficient networks showed a dual response as the
normal and coupling-deficient situation depolarizing currents
reverberating loop stimulation was in this case sufficiently ef-
timed 100 ms apart, of which the second was assumed to be
fective (and 6C, right panels). Thus, when the first
caused by a reverberating loop. In both wild-type and Cx36/
input arrived outside the firing window, the second pulse
mutant cells, the modeled reverberating loop stimulation was
could elicit a response in an uncoupled cell, but not in cou-
in itself, i.e., without the priming induced by the first input, never
pled cells C) (videos in section
strong enough to generate an action potential.
C). Due to the rebound spikes, dual responses, and a wide
The single-cell behavior of the model closely resembled that of
spread in timing of the action potentials in the Cx36-deficient
its biological counterpart as described in the current and previ-
cells, the subthreshold oscillations of ensembles of these cells
ous work (Both cell models oscillate
did not synchronize, despite single-cell resetting effects. As
at 9 Hz, have preferred firing windows on the upward slopes
a result, the variance in timing of these networks' responses
of subthreshold oscillations, exhibit differentially damped oscil-
remained high.
lations after an action potential, and undergo a phase change(resetting effect) upon stimulation (The Cx36-defi-
How May Altered Spiking Patterns Lead
cient cells exhibited subthreshold oscillations with a slightly
to Changes in Learning-Dependent Timing?
larger amplitude than that of the wild-type cells and had an in-
Our data on evoked climbing fiber activities as well as the model
creased chance of generating doublets (12% instead of 0% in
of olivary activities indicate that the temporal firing patterns of oli-
simulated wild-types). Due to dendritic leak currents, the wild-
vary cells are destabilized when they are not coupled. Consider-
type cells were less excitable and had smaller firing windows.
ing that coupling of olivary neurons by Cx36 gap junctions also
In the multiple-cell simulations, electrotonic coupling allowed
synchronizes climbing fiber activities of ensembles of Purkinje
the ensembles of wild-type cells to gradually build up a charge
cells within the same parasagittal zone ;
as pulses come in and to synchronize their action potentials on
but cf. ), one can appreciate that the overall de-
the first input and 6C, left panels) (see also video in
fect in the temporal patterns of complex spike activities within
section C). Still, the coupled wild-type neu-
the olivocerebellar modules must be substantial. To find out
rons did not respond to the reverberant input. In contrast, the
how impaired synchrony of climbing fiber responses may lead
Neuron 58, 599–612, May 22, 2008 ª2008 Elsevier Inc. 605
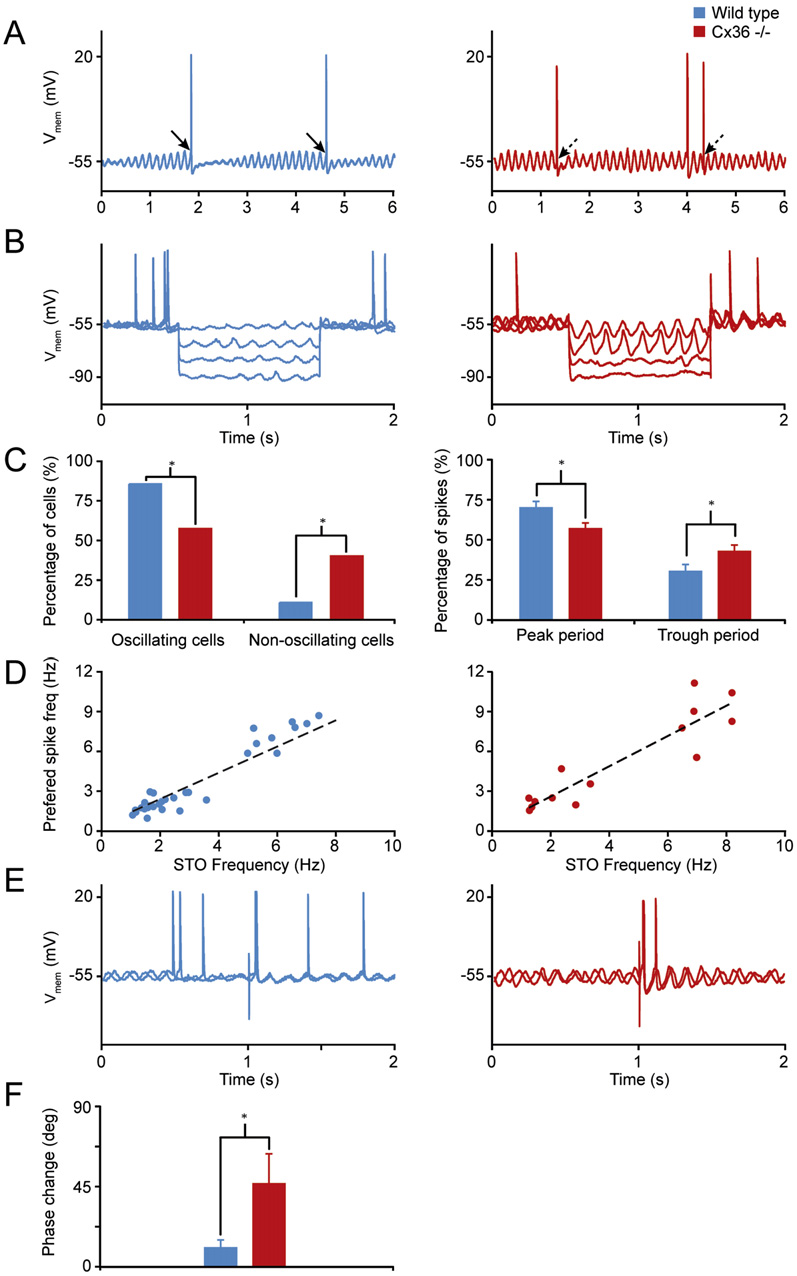
Role of Olivary Coupling in Learning
Figure 5. A Lack of Electrotonic Coupling in the Infe-rior Olive Results in Altered Interactions betweenSubthreshold Oscillations and Generation of SpikingActivities(A) Examples of whole-cell recordings of olivary neurons ina wild-type (blue) and Cx36/ mutant (red) in vivo. Most ofthe olivary spikes in the wild-type occurred around the peakof the oscillations (solid arrows), while those in the mutant fre-quently also occurred in the trough area (dashed arrows).
(B) Subthreshold oscillations also occurred during hyperpola-rizing steps (100 pA) in both wild-types and mutants; however,the oscillations in the mutants depended more strongly on themembrane potential.
(C) (Left panel) Percentages of oscillating and nonoscillatingcells differed significantly among wild-types and Cx36/mutants; *p < 0.01 (c2 test). (Right panel) When oscillating,the percentage of spikes that occurred in the peak periodwas significantly lower in Cx36/ mutants, while that inthe trough period was significantly higher (p < 0.05, t tests).
These periods are defined as the peak amplitude plusor minus 90 and the trough amplitude plus or minus 90,respectively. Percentages indicated are mean values, whileerror bars indicate SD.
(D) Power spectra showed that the frequencies of both theoscillations and spikes occurred in both wild-types and mu-tants mostly in the range from 1 to 3 Hz and from 6 to 9 Hz.
However, the correlation between the preferred frequency ofthe oscillations and that of the spiking activities was signifi-cantly stronger in wild-types (p < 0.01, t test).
(E) Examples of action potentials and subthreshold oscilla-tions in wild-types (left) and Cx36/ mutants (right) beforeand after peripheral stimulation. In both types of animals,the peripheral stimulation had a resetting effect in that theoscillations after the occurrence of the stimulus were inphase with each other, while they were out of phase beforethe oscillations. However, while the phase of the subthresh-old oscillation remained stable in the wild-type, that in themutant was unstable. Stimulus artifacts are set at 1.
(F) The average phase change within the first four cycles often different traces of an individual cell following peripheralstimulation was significantly greater in Cx36/ mutantsthan in wild-types (p < 0.02, t test; error bar indicates SD).
of plasticity in the cerebellar cortex, which is knownto be under climbing fiber control (). We therefore investigated the possibility ofa causal link between absence of properly synchro-nously timed climbing fiber activities and impaired
to deficits in learning-dependent motor timing, we created and
cortical plasticity in a network model of an olivocerebellar mod-
tested a model of the olivocerebellar system controlling condi-
ule. The model initially analyzes the stability of the strengths of
tioned responses and section D).
parallel fiber to Purkinje cell synapses during periods when ac-
The model is in line with the hypothesis that plasticity associated
tive learning is not taking place and when plastic changes of syn-
with learning a conditioned eye-blink response is distributed
aptic strengths may occur due to uncorrelated parallel fiber and
among the cerebellar cortex and cerebellar nuclei, which may
climbing fiber background activity. Subsequently, the impact of
encode the timing of the response and store a representation
synaptic instability is explored in the situation when associative
of the amplitude of the response, respectively (
conditioning occurs, as postlearning (in)stability of parallel fiber
synaptic strength might be directly related to the retention of cor-
). Because a functioning cerebellar cortex has been
tical memory. The following basic assumptions form the guiding
shown to be required for the acquisition and expression of
principles of the model: (1) the olivocerebellar system is topo-
a well-timed conditioned response (the in-
graphically organized, with Purkinje cells, neurons of the cere-
ability of Cx36-deficient mice to learn an appropriately timed
bellar nuclei, and neurons of the inferior olive connected in
eye-blink response might be partly due to incorrect guidance
discrete closed-loop modules in which the Purkinje cells
606 Neuron 58, 599–612, May 22, 2008 ª2008 Elsevier Inc.
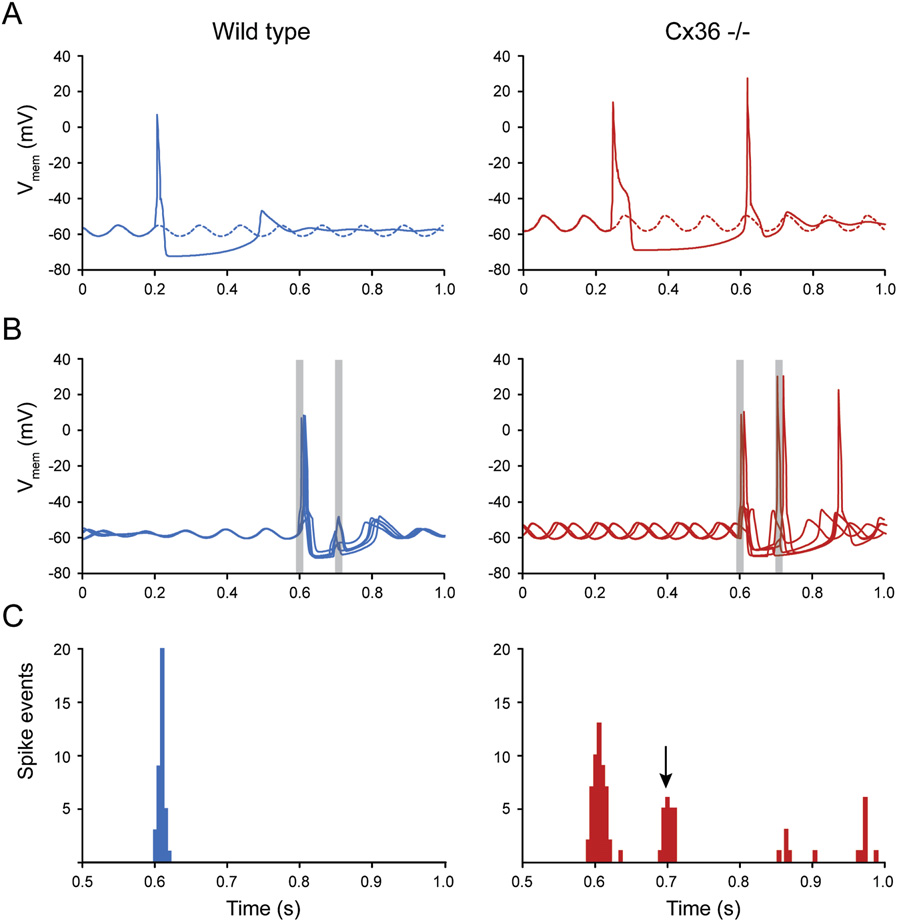
Role of Olivary Coupling in Learning
Figure 6. Simulations of Wild-Type (LeftPanel; Blue) and Cx36 Knockout (RightPanel; Red) Inferior Olivary Cells Using aTwo-Compartmental Computational Model(A) 1000 ms trace of a wild-type cell and a Cx36-deficient cell. Five mA/cm2 depolarizing currentsare applied for 15 ms. The wild-type cell generatesa spike and shows temporarily damped oscilla-tions afterward. The Cx36-deficient cell respondswith a doublet, despite the fact that stimulationoccurred in the trough.
(B) Five traces of 1000 ms from one of the wild-type cell ensemble simulations and a Cx36-defi-cient cell simulation. Due to the random initializa-tion and coupling, the subthreshold oscillationsin the wild-type cells are damped at first andincrease in amplitude as they become more syn-chronized (compare with A). Two depola-rizing 15 ms currents (gray bars) are applied at600 ± 10 ms (SD) (4.5 mA/cm2) and 700 ± 10 ms(SD) (2.5 mA/cm2). Exact onset of the currentswas randomly determined per cell. The spike re-sponses of the wild-type cells occur in a narrowtime window, and the second input does not giverise to any action potentials. Thus, the wild-typenetwork retains its synchronized oscillations. Incontrast, due to a lack of coupling, the Cx36-defi-cient cells do not synchronize their oscillations.
Their spike responses are not timed closely to-gether, the second pulse also gives rise to actionpotentials, and doublets occur. Because of thesefactors, the Cx36-deficient network does notsynchronize.
(C) Temporal distribution of spikes in the wild-typeand Cx36-deficient networks. The first depolariz-ing current was applied at 600 ± 10 ms and the sec-
ond at 700 ± 10 ms. The coupled wild-type networks synchronize the first volley of spikes and do not respond to the second pulse. In the Cx36-deficient network,the first band consists of approximately the same number of spikes as the wild-type response indicated above, but due to a lack of coupling, the spikes are not assynchronized. Because of the increased excitability, Cx36-deficient cells also exhibit responses to the second pulse and an increased number of doublets.
converge onto the nuclei
very same olivary neurons, can stabilize the activities in an entire
((2) the strengths of parallel fiber to Purkinje cell syn-
apses can reversibly increase or decrease, depending, for each
Thus, the model shows how gap junction coupling in the olive
activated granule cell, on the width of the time interval to the
could help to stabilize the weights of parallel fiber to Purkinje cell
climbing fiber stimulus
synapses by synchronizing climbing fiber feedback to Purkinje
and (3) neurons tend to be more active as their excitatory
cells When the activity of olivary neurons is not
inputs increase and less active as their inhibitory inputs increase
synchronized, as is the case in Cx36-deficient mutants, incorrect
feedback through the cerebellar loop causes parallel fiber to Pur-
Based upon these general assumptions, one can deduce that
kinje cell synaptic strengths to drift and finally saturate at their
adaptive changes of synaptic strengths within the olivocerebellar
minimum or maximum strengths (C and 7D). Such
feedback loop can provide feedback to control activity in the in-
impairment in induction and maintenance of cortical plasticity
ferior olive around a stable equilibrium value (
in turn can lead readily to a rectangular ‘‘amplitude'' response
and that such equilibrium in activities of olivary neurons may in
in the nuclei, which prevents an optimal closure of the eyelid at
turn be necessary for synapses in the loop to remain stably fixed
the moment when the unconditioned stimulus is about to take
at their current strength. In the cerebellar loop, it is probably not
place E; compare to A).
directly possible for a Purkinje cell to provide a one-to-one feed-back to its own climbing fiber, due to the strong convergence of
Purkinje cells onto deep cerebellar nuclei neurons A). Toenhance the efficiency of this feedback, specific sets of olivary
Revealing the electrophysiological mechanisms that underlie the
neurons may be selectively coupled. In other words, selective
behavioral phenotypes of Cx36-deficient animals remains a topic
coupling of olivary neurons, which together innervate the com-
of intense investigations (
plete microzone of the Purkinje cells that converge onto the cer-
ebellar nuclei neurons that provide the GABAergic input to the
Neuron 58, 599–612, May 22, 2008 ª2008 Elsevier Inc. 607
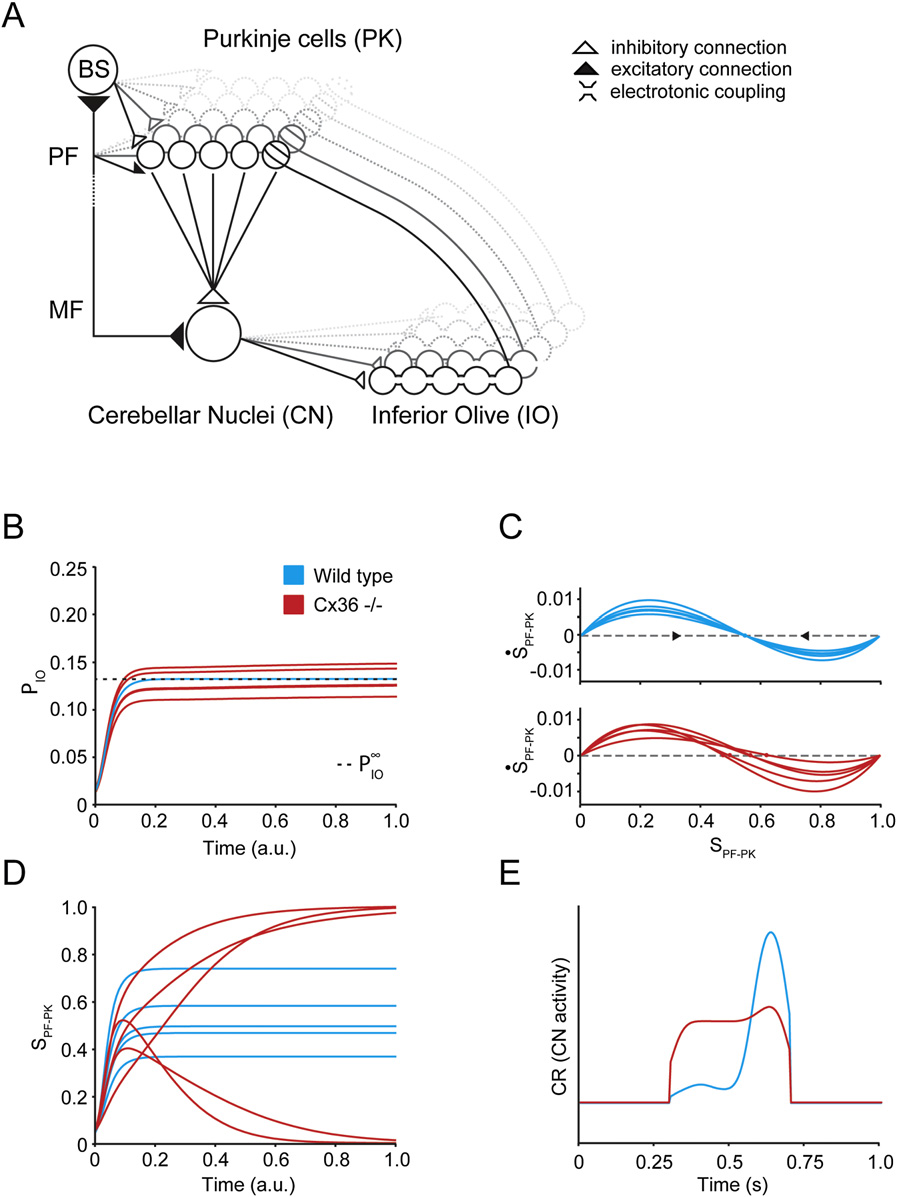
Role of Olivary Coupling in Learning
Figure 7. Impact of Altered Olivary Couplingand Spiking on Learning-Dependent Timingin a Network SimulationFor panels (A)–(D), a mathematical model wasused to analyze the stability of parallel fiber to Pur-kinje cell synapses as a function of the averagebackground spike probabilities of parallel fibersand climbing fibers. Panel (E) displays resultsfrom a real-time simulation, which incorporatesthe findings of the mathematical model in a condi-tioning paradigm. In the simulations, synapticweights are adjusted according to coincidencerules for parallel fiber and climbing fiber activity.
Neural spike probabilities are represented as lin-ear sums of inhibitory and excitatory synapticinputs, which are defined as the product of pre-synaptic average activity and synaptic strength.
(A) Connectivity within a cerebellar module; Pur-kinje cells (PK), neurons of the cerebellar nuclei(CN), and neurons of the inferior olive (IO) forma closed loop. A key feature is the strong conver-gence of Purkinje cells onto CN neurons. MF, PF,and BS indicate mossy fibers, parallel fibers, andbasket cell/stellate cells, respectively. (B) Measurefor the spike probability of five IO neurons as afunction of time. Two different situations are inves-tigated: a synchronized IO, in which IO neuronsactivate collectively due to coupling (wild-types,blue), and an asynchronous IO, in which each neu-ron fires independently (Cx36/ mutants, red).
Wild-type curves all settle at the same equilibriumvalue PN, while mutant curves remain above or
below this value. (C) Phase plots of the averagePF to PK synaptic strength S of five Purkinje cells.
The derivative of S represents the plastic changeof S at each time step due to synaptic plasticity.
PF activity determines the rate at which plasticityoccurs, while the ratio of depression versus poten-tiation depends on the frequency of climbing fiberactivation. Synaptic strengths are stable when thederivative of S equals zero. (Top panel) A synchro-nous IO produces synchronous climbing fiberactivation, such that all Purkinje cells share a com-mon stable state. (Bottom panel) In an asynchro-nous IO, each neuron fires at its own specificrate, resulting in different depression versuspotentiation ratios in each Purkinje cell. Thus, inthis situation each individual Purkinje cell strivesfor a different state of the network in order to sta-
bilize its synaptic strengths. (D) Development of synaptic strengths in time for a synchronous IO (blue) and an asynchronous IO (red). Wild-type Purkinje cells allreceive the same stabilizing climbing fiber input, at which depression and potentiation exactly cancel each other over time. Their synaptic strengths thereforeremain stable. Cx36/ mutant Purkinje cells receive climbing fiber inputs that are either above or below equilibrium, resulting in too much depression or poten-tiation, respectively. Synapses therefore saturate at their minimum or maximum strengths. (E) Eye-blink responses of wild-types (blue) and Cx36/ mutants (red)resulting from a real-time simulation of the architecture in panel (A). An additional plasticity rule was incorporated at the MF to CN synapse, allowing for the de-velopment of the ‘‘block'' response in the CN. In wild-types, inhibitory input from the cortex modulates the ‘‘block'' activity in the CN to produce a well-timedresponse. In Cx36/ mutants, saturation of PF to PK synaptic strengths prevents induction and maintenance of the right levels of depression and potentiation,resulting in insufficient cortical modulation. Details are provided as (part D).
). Here, we show that a lack of electrotonic cou-
tioned responses occurred, but the timing that had to be learned
pling in the inferior olive leads to abnormal firing patterns of its
to make the response optimal was aberrant. In the locomotion
neurons, which in turn can contribute to deficits in the timing of
test on the Erasmus ladder, Cx36/ mutants were impaired in
conditioned motor responses. The behavioral deficits in the
learning to avoid a bar that rose after a fixed period after the
global Cx36/ mutants could be prominently revealed in differ-
onset of the tone. Likewise, in the eye-blink test, the coupling-
ent paradigms in which either a locomotion response or an eye-
deficient mutants showed after four training sessions a mismatch
blink response was conditioned to a tone. In both cases, condi-
in their latency to peak amplitude of 200 ms with respect to the
608 Neuron 58, 599–612, May 22, 2008 ª2008 Elsevier Inc.
Role of Olivary Coupling in Learning
onset of an air puff. This period stands in marked contrast to the
Thus, together with the current findings, we conclude that cou-
10–20 ms delay that can be observed in the basic eye movement
pling supports the oscillations in the inferior olive in vivo and
responses of Cx36 null mutants to optokinetic stimulation (
that the impact of oscillations on spiking patterns is reduced in
) or in the tremorgenic, limb, and body movements of
uncoupled olivary neurons, which results in a more random tim-
coupling-deficient rats treated with replication-incompetent len-
ing of their spikes in relation to the oscillations.
tiviral vectors (The behavioral deficits
The question remains as to how dynamic regulation of electro-
in learning-dependent timing in the Cx36/ mutants were
tonic coupling in the olive may contribute to learning-dependent
robust despite secondary compensations that may occur
(Moreover, we observed the same type
of deficits in the conditioning process following application of
and colleagues have provided strong evidence that the GABAer-
carbenoxolone and mefloquine, which are known to block olivary
gic input from the cerebellar nuclei, which terminates strategically
coupling in an acute fashion (
at the coupled dendrites in olivary glomeruli (
), can uncouple the olivary neurons (
cannot rule out short-term compensations or side effects with
). According to our model, such a mecha-
this approach either
nism would counteract learning-dependent timing of the condi-
still the behavioral effects were significant and similar
tioned responses, because it desynchronizes the climbing fiber
to those in the global mutants, while they cannot be due to
inputs that mediate the signals of the US. Interestingly, Mauk
the potential long-term compensations that may occur in the
and colleagues recently demonstrated that the GABAergic pro-
Cx36/ mutants. Importantly, the effects of mefloquine could
jection to the olive is necessary for extinction of the conditioned
be readily observed after injection into the inferior olive itself, in-
responses by reducing the firing rate of the relevant olivary neu-
dicating that it is the coupling in the olive that is essential for learn-
rons below resting level (). Thus, in this respect,
ing-dependent timing of motor responses. These data in turn
the mechanism of uncoupling and the mechanism of reducing the
were supported by the finding that the abnormal timing of the
firing rate of olivary neurons may serve the same effect in that they
conditioned eye-blink responses in the global Cx36/ mutants
both actively counteract the conditioning process.
was not observed in floxed Cx36 knockouts, in which Cx36 is nor-mally expressed in the relevant olivary subnuclei, but not in most
EXPERIMENTAL PROCEDURES
other Cx36-expressing neurons in the brain. Together, these find-ings indicate that the role of coupling between inferior olivary
Global Cx36/ mutants and wild-type mice were generated and character-
neurons becomes apparent when the olivocerebellar system is
ized as described by The mutants that lacked Cx36
challenged in a cerebellar motor learning task and that it serves
in their cerebellar nuclei neurons were cross-breedings of floxed-Cx36 mu-
to facilitate learning-dependent timing in response to unexpected
tants and parvalbumin-Cre mice. In contrast to the mouse published in
events rather than timing of movements per se.
the current conditional Cx36-deficient mouse has the 50 loxP site
In combination with modeling studies of both olivary neurons
inserted in the 50UTR of Cx36 and not in the intron, leading to functional
and the olivocerebellar system as a whole, our electrophysiolog-
expression of the floxed Cx36 allele. Furthermore, after Cre-mediated deletion,a cyan fluorescent protein (CFP) was expressed instead of the floxed Cx36
ical recordings demonstrated that a lack of precision in the tim-
allele (B). Transfection of embryonic stem cells, creation of cell
ing of the climbing fiber responses mediating the US signals
cultures, screening for cell clones, and injections of blastocysts were per-
(present data) as well as a reduction in synchrony of climbing
formed as described (). Subsequently, Cx36+/flox(CFP)
fiber activities during spontaneous activity (
mice were mated to Cx36+/del (lacZ): parvalbumin-Cre mice to obtain cerebel-
but cf. are probably responsible for
lum-deleted offspring with the genotype Cx36 del(lacZ)/flox(CFP): parvalbu-
the behavioral deficits in the coupling-deficient Cx36/
min-Cre. For this study, we used offspring from a parvalbumin-Cre founder
mutants. The lack of precision in timing is due to increased vari-
with multicopy integration of the transgene. All experiments were conductedin accordance with the European Communities Council Directive (86/609/
ety in the responses after the US, which in turn probably results
EEC) and approved by the Dutch, British, and/or German national ethics
from altered interactions with the subthreshold oscillations in the
inferior olive of Cx36/ mutants. Our whole-cell recordings ofolivary neurons in vivo did not only demonstrate that Cx36/
mutants show less frequently subthreshold oscillations and
Cx36-/flox: parvalbumin-Cre mice and Cx36-/flox(CFP) littermate controls
that the stability of the remaining oscillations is reduced but
were anesthetized and perfused with 4% paraformaldehyde in PBS. Thebrains were removed and cut on a vibratome (Leica, UK), and the sections
also that the incidence of spikes occurring in the trough of these
were immersed in rabbit anti-GFP (1:1000, Invitrogen, UK). Sections were
oscillations is significantly higher, while the correlation between
washed in PBS and incubated in donkey anti-rabbit Cy3 (1:1000, Jackson
their preferred spiking pattern and the frequency of their oscilla-
ImmunoResearch, USA) prior to mounting (Vector Labs, UK). Cy3 was viewed
tions is generally weaker. The robust appearance of subthresh-
with a custom filter set and Alexa 488 with FITC filters (Nikon E600 micro-
old oscillations of olivary neurons has recently also been shown
scope), and images were captured directly from the slide using an Acquis
in vivo in rats with the use of sharp electrode recordings
Image Capture system (Synoptics, UK).
), while the contribution of electrotonic coupling to
the occurrence of subthreshold oscillations in olivary cells has
The Erasmus ladder is a fully automated system to screen both motor perfor-
recently also been addressed in rats with the use of lentiviral
mance and motor learning capabilities of mutant mice in a noninvasive manner
knockdown of Cx36 or pharmacological blockage of gap junc-
at a high-throughput level (for details see section A). It con-
sists of a horizontal ladder in between two shelter boxes, which are equipped
Neuron 58, 599–612, May 22, 2008 ª2008 Elsevier Inc. 609
Role of Olivary Coupling in Learning
with two pressurized air outlets (Pneumax, 171E2B.T.A.0009) to control the
Simulations of Inferior Olive
moment of departure and speed of the mouse. The ladder has 2 3 37 rungs
The model used for the inferior olivary cell ensemble simulations was a modi-
for the left and right side. All rungs are equipped with pressure sensors (pro-
fied version of a two-compartmental cell model by
duced at Erasmus MC), which are continuously monitored and which can be
The h current was moved to the dendritic compartment and redefined as:
used to register and analyze the walking pattern of the mouse instantaneously.
Moreover, based upon the prediction of the walking pattern, the rungs can be
qNðVdÞ =
moved up or down by a high-speed pneumatic slide (Pneumax, 2141.
1 + exp½ðVd + 80Þ=4
52.00.36.91) with a maximum of 13 mm at any moment in time. The computersystem (National Instruments, PXI-1000B) that runs the real-time system to
record sensor data, adjust air pressure, predict future touches, calculate
tqðVdÞ = expð 0:086Vd 14:6Þ + expð0:07Vd 1:87Þ
interventions, reposition slides, and store data operates in a fixed cycle of2 ms. In the conditioning procedures, a 15 kHz tone (Voltcraft 7202), which
gradually increases over 20 ms to 100 dB and which lasts up to 300 ms,
d as the dendritic membrane potential. Per cell type, five ensembles of
neurons on a 5 3 5 grid were simulated. Wild-type cells were connected to
was used as the CS, while a rising rung, which ascends 12 mm, was used
all directly neighboring neurons (up to 8) with gap junction conductance set
as the US. The trials were separated by a random intertrial interval ranging
at 0.04 pS/cm2. For the mutant model, Ca2+ conductances were increased
from 8 to 12 s.
as suggested by earlier research (), with the new conduc-
Pharmacological interventions were done with either carbenoxolone injec-
tances for high- and low-threshold Ca2+ set at 4.4 pS/cm2 and 1.3 pS/cm2,
tions i.p. (40 mg/kg in 0.9% NaCl) or mefloquine injections into the olive
respectively (a 10% increase compared to the wild-type values). Of the two
(150 mM in 0.2% DMSO). Dosages were chosen so as to make sure that impact
depolarizing currents used, the first (4.5 mA/cm2, 15 ms) was applied at
on coupling was warranted, while side effects were minimized (
600 ± 10 ms after the start of the simulation, and the second (2.5 mA/cm2,
15 ms) at 700 ± 10 ms. The exact timing was randomly determined per cell.
Mice were anesthetized with ketamine/xylazine (80 mg/kgand 2 mg/kg), their atlanto-occipital membrane was opened, and the olive
Model and Simulations of Olivocerebellar System
was identified electrophysiologically ). Sub-
In the model, variable synaptic strengths are governed by coincidence rules for
sequently, the recording pipette was replaced by a pipette (tip diameter:
parallel fiber and climbing fiber activity probabilities. Synapses are accordingly
10–12 mm) filled with mefloquine and/or 2% BDA (Molecular Probes, Leiden,
described by differential equations, which are coupled by linear relations that
The Netherlands), and pressure injections of 50–100 nl were made within the
represent the olivocerebellar loop. This formulation directly allows producing
olivary complex (see C). After the conditioning sessions, the animals
the phase plots shown in The equations were solved by numerical
were anesthetized with an overdose of pentobarbital (200 mg/kg i.p.) and per-
integration using MATLAB's function ode45, which is a fourth-order Runge-
fused with saline and paraformaldehyde (4% in phosphate buffer). The brains
Kutta solver. Resulting time-trajectory plots of synaptic strengths are shown
were collected, embedded in gelatin, cut at 40 mm transverse sections, and
in D. The model equations were then implemented in real-time simu-
mounted (In case of the BDA injections, sections
lations running with a 5 ms time-step (performed in MATLAB). In these real-
were processed according to a BDA protocol using ABC-elite (Vector Labora-
time simulations, inferior olivary neurons were simulated as spiking neurons.
tories) and DAB histochemistry
A training paradigm consisting of paired inputs representing conditioned stim-ulus and unconditioned stimulus was used to train the network, both in thecase of synchronous and asynchronous inferior olive activity (Fur-
Eye-Blink Conditioning
ther details on constraints, formulas, and simulations are presented in an
Wild-type and mutant mice were prepared for eye-blink conditioning accord-
extensive report in the (section D).
ing to the MDMT procedure (). Mice were sub-jected to either a paired or a randomly paired procedure; both procedureslasted 4 days, during each of which one session (64 trials grouped in 8 blocks)
SUPPLEMENTAL DATA
was conducted.
The Supplemental Data for this article can be found online at .
Extracellular Recordings
Mice were prepared by placing a recording chamber above the simplex lobule
and adjacent areas. Extracellular Purkinje cell activities were recorded in theeye-blink region of awake animals with glass microelectrodes using a Multi-
We thank E. Dalm and J.v.d. Burg for their technical assistance, R. Maex for his
clamp 700A and Digidata 1322A from Axon Instruments. Complex spike re-
advise on the model, and T.J.H. Ruigrok for help with the injections. The work
sponses were recorded during 1 Hz air puff stimulation to the eye and analyzed
in the group of C.I.D.Z. was supported by the Dutch Organization for Medical
off-line. A voltage threshold was used to detect complex spikes, and the time
Sciences (ZON-MW), Life Sciences (NWO-ALW), Senter (Neuro-Bsik), Prinses
and waveform of the voltage records were used for off-line analysis (IGOR
Beatrix Fonds, and the European Community (EEC; SENSOPAC). Work in the
analysis software; WaveMetrics, OR).
Bonn laboratory was supported by the German Research Association (Wi 270/22-5,6) to K.W. Research of the H.M. team is supported by the SchillingFoundation.
Whole-Cell Recordings In Vivo
Mice were prepared for experiments under isoflurane or ketamine/xylazine
Received: July 16, 2007
anesthesia (for details see Recordings were amplified
Revised: December 12, 2007
with a MultiClamp 700A and DIGIDATA 1322A (Axon Instruments), and mem-
Accepted: March 9, 2008
brane potentials were corrected for junction potential (8 mV). In current-clamp
Published: May 21, 2008
experiments, we measured voltage responses to a series of negative currentsteps (100 pA). Correlations between the subthreshold oscillation frequencies
and preferred spiking frequencies were determined by analyzing autocorrelo-grams (clampfit 9.0 software, Axon Instruments, CA). Bursts with a significant
Bengtsson, F., and Hesslow, G. (2006). Cerebellar control of the inferior olive.
poisson surprise value (>5) were correlated to the corresponding subthreshold
Cerebellum 5, 7–14.
oscillation (STO) frequency extracted from the power spectrum of the
Bennett, M.V., and Zukin, R.S. (2004). Electrical coupling and neuronal
synchronization in the Mammalian brain. Neuron 41, 495–511.
610 Neuron 58, 599–612, May 22, 2008 ª2008 Elsevier Inc.
Role of Olivary Coupling in Learning
Blenkinsop, T.A., and Lang, E.J. (2006). Block of inferior olive gap junctional
Hesslow, G. (1994). Correspondence between climbing fibre input and motor
coupling decreases Purkinje cell complex spike synchrony and rhythmicity.
output in eyeblink-related areas in cat cerebellar cortex. J. Physiol. 476, 229–
J. Neurosci. 26, 1739–1748.
Buhl, D.L., Harris, K.D., Hormuzdi, S.G., Monyer, H., and Buzsaki, G. (2003).
Hesslow, G., Svensson, P., and Ivarsson, M. (1999). Learned movements
Selective impairment of hippocampal gamma oscillations in connexin-36
elicited by direct stimulation of cerebellar mossy fiber afferents. Neuron
knock-out mouse in vivo. J. Neurosci. 23, 1013–1018.
Bullock, T.H., Bennett, M.V., Johnston, D., Josephson, R., Marder, E., and
Kenyon, G.T., Medina, J.F., and Mauk, M.D. (1998). A mathematical model of
Fields, R.D. (2005). Neuroscience. The neuron doctrine, redux. Science 310,
the cerebellar-olivary system I: self-regulating equilibrium of climbing fiber ac-
tivity. J. Comput. Neurosci. 5, 17–33.
Chorev, E., Yarom, Y., and Lampl, I. (2007). Rhythmic episodes of subthresh-
Kistler, W.M., De Jeu, M.T., Elgersma, Y., Van Der Giessen, R.S., Hensbroek,
old membrane potential oscillations in the rat inferior olive nuclei in vivo.
R., Luo, C., Koekkoek, S.K., Hoogenraad, C.C., Hamers, F.P., Gueldenagel,
J. Neurosci. 27, 5043–5052.
M., et al. (2002). Analysis of Cx36 knockout does not support tenet that olivary
Coesmans, M., Weber, J.T., De Zeeuw, C.I., and Hansel, C. (2004). Bidirec-
gap junctions are required for complex spike synchronization and normal mo-
tional parallel fiber plasticity in the cerebellum under climbing fiber control.
tor performance. Ann. N Y Acad. Sci. 978, 391–404.
Neuron 44, 691–700.
Khosrovani, S., Van Der Giessen, R.S., De Zeeuw, C.I., and De Jeu, M.T.
Condorelli, D.F., Parenti, R., Spinella, F., Trovato Salinaro, A., Belluardo, N.,
(2007). In vivo mouse inferior olive neurons exhibit heterogeneous subthresh-
Cardile, V., and Cicirata, F. (1998). Cloning of a new gap junction gene
old oscillations and spiking patterns. Proc. Natl. Acad. Sci. USA 104, 15911–
(Cx36) highly expressed in mammalian brain neurons. Eur. J. Neurosci. 10,
Koekkoek, S.K., Den Ouden, W.L., Perry, G., Highstein, S.M., and De Zeeuw,
Connors, B.W., and Long, M.A. (2004). Electrical synapses in the mammalian
C.I. (2002). Monitoring kinetic and frequency-domain properties of eyelid
brain. Annu. Rev. Neurosci. 27, 393–418.
responses in mice with magnetic distance measurement technique. J. Neuro-physiol. 88, 2124–2133.
Cruikshank, S.J., Hopperstad, M., Younger, M., Connors, B.W., Spray, D.C.,and Srinivas, M. (2004). Potent block of Cx36 and Cx50 gap junction channels
Koekkoek, S.K., Hulscher, H.C., Dortland, B.R., Hensbroek, R.A., Elgersma,
by mefloquine. Proc. Natl. Acad. Sci. USA 101, 12364–12369.
Y., Ruigrok, T.J., and De Zeeuw, C.I. (2003). Cerebellar LTD and learning-dependent timing of conditioned eyelid responses. Science 301, 1736–1739.
De Zeeuw, C.I., and Yeo, C.H. (2005). Time and tide in cerebellar memory for-mation. Curr. Opin. Neurobiol. 15, 667–674.
Koekkoek, S.K., Yamaguchi, K., Milojkovic, B.A., Dortland, B.R., Ruigrok, T.J.,Maex, R., De Graaf, W., Smit, A.E., VanderWerf, F., Bakker, C.E., et al. (2005).
De Zeeuw, C.I., Hertzberg, E.L., and Mugnaini, E. (1995). The dendritic lamellar
Deletion of FMR1 in Purkinje cells enhances parallel fiber LTD, enlarges spines,
body: a new neuronal organelle putatively associated with dendrodendritic
and attenuates cerebellar eyelid conditioning in Fragile X syndrome. Neuron
gap junctions. J. Neurosci. 15, 1587–1604.
De Zeeuw, C.I., Simpson, J.I., Hoogenraad, C.C., Galjart, N., Koekkoek, S.K.,
Landisman, C.E., Long, M.A., Beierlein, M., Deans, M.R., Paul, D.L., and Con-
and Ruigrok, T.J. (1998). Microcircuitry and function of the inferior olive. Trends
nors, B.W. (2002). Electrical synapses in the thalamic reticular nucleus. J. Neu-
Neurosci. 21, 391–400.
rosci. 22, 1002–1009.
De Zeeuw, C.I., Chorev, E., Devor, A., Manor, Y., Van Der Giessen, R.S., De
Lang, E.J., Sugihara, I., and Llinas, R. (1996). GABAergic modulation of
Jeu, M.T., Hoogenraad, C.C., Bijman, J., Ruigrok, T.J., French, P., et al.
complex spike activity by the cerebellar nucleoolivary pathway in rat. J. Neuro-
(2003). Deformation of network connectivity in the inferior olive of connexin
physiol. 76, 255–275.
36-deficient mice is compensated by morphological and electrophysiologicalchanges at the single neuron level. J. Neurosci. 23, 4700–4711.
Leznik, E., and Llinas, R. (2005). Role of gap junctions in synchronized neuronaloscillations in the inferior olive. J. Neurophysiol. 94, 2447–2456.
Deans, M.R., Gibson, J.R., Sellitto, C., Connors, B.W., and Paul, D.L. (2001).
Synchronous activity of inhibitory networks in neocortex requires electrical
Leznik, E., Makarenko, V., and Llinas, R. (2002). Electrotonically mediated
synapses containing connexin36. Neuron 31, 477–485.
oscillatory patterns in neuronal ensembles: an in vitro voltage-dependentdye-imaging study in the inferior olive. J. Neurosci. 22, 2804–2815.
Deans, M.R., Volgyi, B., Goodenough, D.A., Bloomfield, S.A., and Paul, D.L.
(2002). Connexin36 is essential for transmission of rod-mediated visual signals
Llinas, R., and Yarom, Y. (1981). Electrophysiology of mammalian inferior oli-
in the mammalian retina. Neuron 36, 703–712.
vary neurones in vitro. Different types of voltage-dependent ionic conduc-tances. J. Physiol. 315, 549–567.
Degen, J., Meier, C., Van Der Giessen, R.S., Sohl, G., Petrasch-Parwez, E.,Urschel, S., Dermietzel, R., Schilling, K., De Zeeuw, C.I., and Willecke, K.
Llinas, R., and Sasaki, K. (1989). The functional organization of the olivo-cere-
(2004). Expression pattern of lacZ reporter gene representing connexin36 in
bellar system as examined by multiple purkinje cell recordings. Eur. J. Neuro-
transgenic mice. J. Comp. Neurol. 473, 511–525.
sci. 1, 587–602.
Frisch, C., De Souza-Silva, M.A., Sohl, G., Guldenagel, M., Willecke, K., Hus-
Long, M.A., Deans, M.R., Paul, D.L., and Connors, B.W. (2002). Rhythmicity
ton, J.P., and Dere, E. (2005). Stimulus complexity dependent memory impair-
without synchrony in the electrically uncoupled inferior olive. J. Neurosci. 22,
ment and changes in motor performance after deletion of the neuronal gap
junction protein connexin36 in mice. Behav. Brain Res. 157, 177–185.
Long, M.A., Jutras, M.J., Connors, B.W., and Burwell, R.D. (2005). Electrical
Garcia, K.S., and Mauk, M.D. (1998). Pharmacological analysis of cerebellar
synapses coordinate activity in the suprachiasmatic nucleus. Nat. Neurosci.
contributions to the timing and expression of conditioned eyelid responses.
8, 61–66.
Neuropharmacology 37, 471–480.
Mann-Metzer, P., and Yarom, Y. (1999). Electrotonic coupling interacts with
Gareri, P., Condorelli, D., Belluardo, N., Citraro, R., Barresi, V., Trovato-Sali-
intrinsic properties to generate synchronized activity in cerebellar networks
nato, A., Mudo , G., Ibbadu, G.F., Russo, E., and De Sarro, G. (2005). Antiab-
of inhibitory interneurons. J. Neurosci. 19, 3298–3306.
sence effects of carbenoxolone in two genetic animal models of absence
Margineanu, D.G., and Klitgaard, H. (2006). The connexin 36 blockers quinine,
epilepsy (WAG/Rij rats and lh/lh mice). Neuropharmacology 49, 551–563.
quinidine and mefloquine inhibit cortical spreading depression in a rat neocor-
Guldenagel, M., Ammermuller, J., Feigenspan, A., Teubner, B., Degen, J.,
tical slice model in vitro. Brain Res. Bull. 71, 23–28.
Sohl, G., Willecke, K., and Weiler, R. (2001). Visual transmission deficits in
Marshall, S.P., Van Der Giessen, R.S., De Zeeuw, C.I., and Lang, E.J. (2007).
mice with targeted disruption of the gap junction gene connexin36. J. Neuro-
Altered olivocerebellar activity patterns in the connexin36 knockout mouse.
sci. 21, 6036–6044.
Cerebellum 6, 287–299.
Neuron 58, 599–612, May 22, 2008 ª2008 Elsevier Inc. 611
Role of Olivary Coupling in Learning
Martin, F.C., and Handforth, A. (2006). Carbenoxolone and mefloquine sup-
Ruigrok, T.J.H., and Voogd, J. (1995). Cerebellar influence on olivary excitabil-
press tremor in the harmaline mouse model of essential tremor. Mov. Disord.
ity in the cat. Eur. J. Neurosci. 7, 679–693.
Ruigrok, T.J., and Voogd, J. (2000). Organization of projections from the infe-
Mauk, M.D., and Donegan, N.H. (1997). A model of Pavlovian eyelid condition-
rior olive to the cerebellar nuclei in the rat. J. Comp. Neurol. 426, 209–228.
ing based on the synaptic organization of the cerebellum. Learn. Mem. 4, 130–158.
Ruigrok, T.J., and Apps, R. (2007). A light microscope-based double retro-grade tracer strategy to chart central neuronal connections. Nat. Protocols
Mauk, M.D., Steinmetz, J.E., and Thompson, R.F. (1986). Classical condition-
ing using stimulation of the inferior olive as the unconditioned stimulus. Proc.
Natl. Acad. Sci. USA 83, 5349–5353.
Saffitz, J.E., Laing, J.G., and Yamada, K.A. (2000). Connexin expression and
Medina, J.F., and Mauk, M.D. (2000). Computer simulation of cerebellar infor-
turnover: implications for cardiac excitability. Circ. Res. 86, 723–728.
mation processing. Nat. Neurosci. Suppl. 3, 1205–1211.
Schweighofer, N., Doya, K., and Kawato, M. (1999). Electrophysiological prop-
Medina, J.F., Nores, W.L., and Mauk, M.D. (2002). Inhibition of climbing
erties of inferior olive neurons: A compartmental model. J. Neurophysiol. 82,
fibres is a signal for the extinction of conditioned eyelid responses. Nature
Seto-Ohshima, A., Emson, P.C., Berchtold, M.W., and Heizmann, C.W. (1989).
Perrett, S.P., Ruiz, B.P., and Mauk, M.D. (1993). Cerebellar cortex lesions dis-
Localization of parvalbumin mRNA in rat brain by in situ hybridization histo-
rupt learning-dependent timing of conditioned eyelid responses. J. Neurosci.
chemistry. Exp. Brain Res. 75, 653–658.
Simpson, J.I., Wylie, D.R., and De Zeeuw, C.I. (1996). On climbing fiber signals
Pijpers, A., Voogd, J., and Ruigrok, T.J. (2005). Topography of olivo-cortico-
and their consequences. Behav. Brain Sci. 19, 380–395.
nuclear modules in the intermediate cerebellum of the rat. J. Comp. Neurol.
492, 193–213.
Sohl, G., Degen, J., Teubner, B., and Willecke, K. (1998). The murine gap junc-tion gene connexin36 is highly expressed in mouse retina and regulated during
Placantonakis, D.G., Bukovsky, A.A., Zeng, X.H., Kiem, H.P., and Welsh, J.P.
brain development. FEBS Lett. 428, 27–31.
(2004). Fundamental role of inferior olive connexin 36 in muscle coherenceduring tremor. Proc. Natl. Acad. Sci. USA 101, 7164–7169.
Theis, M., Magin, T.M., Plum, A., and Willecke, K. (2000). General or cell type-
Placantonakis, D.G., Bukovsky, A.A., Aicher, S.A., Kiem, H.P., and Welsh, J.P.
specific deletion and replacement of connexin-coding DNA in the mouse.
(2006). Continuous electrical oscillations emerge from a coupled network:
Methods 20, 205–218.
a study of the inferior olive using lentiviral knockdown of connexin36. J. Neuro-
Van Alphen, A.M., Schepers, T., Luo, C., and De Zeeuw, C.I. (2002). Motor
sci. 26, 5008–5016.
performance and motor learning in Lurcher mice. Ann. N Y Acad. Sci. 978,
Porras-Garcia, E., Cendelin, J., Dominguez-del-Toro, E., Vozeh, F., and Del-
gado-Garcia, J.M. (2005). Purkinje cell loss affects differentially the execution,
Van Der Giessen, R.S., Maxeiner, S., French, P.J., Willecke, K., and De Zeeuw,
acquisition and prepulse inhibition of skeletal and facial motor responses in
C.I. (2006). Spatiotemporal distribution of Connexin45 in the olivocerebellar
Lurcher mice. Eur. J. Neurosci. 21, 979–988.
system. J. Comp. Neurol. 495, 173–184.
Raymond, J.L., Lisberger, S.G., and Mauk, M.D. (1996). The cerebellum: a neu-ronal learning machine? Science 272, 1126–1131.
Voogd, J., and Glickstein, M. (1998). The anatomy of the cerebellum. TrendsNeurosci. 21, 370–375.
Rozental, R., Srinivas, M., and Spray, D.C. (2001). How to close a gap junctionchannel. Efficacies and potencies of uncoupling agents. Methods Mol. Biol.
Wang, S.S., Denk, W., and Hausser, M. (2000). Coincidence detection in single
dendritic spines mediated by calcium release. Nat. Neurosci. 3, 1266–1273.
612 Neuron 58, 599–612, May 22, 2008 ª2008 Elsevier Inc.
Source: http://teachline.ls.huji.ac.il/72369/YaromsPDFs/IO/DeZeeuw_timing_olive_coupling.pdf
Table des matières PATHOLOGIE RHINO-SINUSIENNE AIGUË .2 Rhinites aiguës.2 Sinusites aiguës .2 L'ethmoïdite aiguë de l'enfant .5 PATHOLOGIE RHINO-SINUSIENNE CHRONIQUE.7 Classification des pathologies rhinosinusiennes chroniques .7 Diagnostic de rhinosinusites chronique .7 Les sinusites antérieures de la face.7 Les pan-sinusites bilatérales et symétriques .8
Case reports of Acne with long lasting follow up. Chatzimanuil A. DDS Secretary Dernikou M.F. MD, MSc Homeopathy. Tsilikas A.MD, Dermatologist, MSc Homeopathy,board member Michalakis M. DDS, MSc, specialist in Oral Diagnostics&Radiology,President. Some think of acne as a modern phenomenon. The word "aku-t" occurs in the Ebers Papyrus 6